© Springer-Verlag London Ltd. 2017
Jack L. Arbiser (ed.)Angiogenesis-Based Dermatology10.1007/978-1-4471-7314-4_22. Infantile Hemangioma: New Insights on Pathogenesis and Beta Blockers Mechanisms of Action
(1)
The Department of Dermatology and Sheba Cancer Research Center, Sheba Medical Center, Ramat-Gan, 52621, Israel
Keywords
Infantile HemangiomaBeta BlockersPropranololAngiogenesisVasculogenesisHemangioma Stem CellsIntroduction
Infantile hemangioma (IH) is the most common tumor of infancy, affecting 5–10% of infants at the end of the first year. Increased risk factors for IH include Caucasian race, female gender (4:1 female to male ratio) [1], prematurity, low birth weight, and being the product of multiple gestations [2]. There is increased risk in premature neonates under the weight of 1,500 g, in females and in Caucasians. The superficial tumors are usually noticed initially in the first 4 weeks after birth as brightly red macule, papule or plaque. In contrast, deep-seated IHs often present later, at 2–3 months of age, as soft blue nodules.
Clinical
IH is a benign tumor, and in most patients it carries no risk of morbidity or mortality. However, in 10% of patients IH is problematic or even endangering to the child, due to its anatomical location or due to excessive growth. The great majority of IH are focal and solitary. Sixty percent of the tumors occur on the head and neck, 25% on the trunk, and 15% on the extremities. The tumor displays a unique life cycle that can be separated clinically into three phases [3, 4]. The proliferating phase starts within first weeks of life and ends within the first year of life, with the most growth occurring during the first 4–6 months of life. At this phase, the tumor changes its presentation from mild blanching, fine telangiectasias or a red or macule to a prominent bright red papule, plaque or nodule [5]. The involution phase begins on average at 12 months of age. The tumor changes color from bright red to less red and gray. Also, nodules and papules shrink and soften. Finally, at the involuted phase, tumor growth has stopped and the tumor regressed. About half of tumors involute by age 5 years and 70% by age 7 years. In 30%, an additional 3–5 years to complete the process [6]. The regression of IH often leaves residues. These residues are correlated to the maximum size of the hemangioma. These include telangiectasias, atrophic, wrinkled skin, hyper or hypopigmentation as well as redundant fibrofatty tissue [7].
Histopathology and Cellular Components
The pathological phases of IH strongly correlate with the clinical phases. The proliferating lesions display solid, cellular lobules consisting of plump endothelial cells lining tiny rounded vascular spaces with inconspicuous lumina. In the involuting phase the vascular channels contain flattened, mature, endothelial cells, an organized perivascular layer and basement membrane. Apoptotic cells, at least third of them endothelial are seen [8–10] and an increase in the number of mast cells. Lastly, at the involuted phase, fat, fibroblasts and connective tissue replace the vascular tissue, with few remaining large feeding and draining vessels evident.
In recent years, several cellular components of IH were isolated and characterized. These include hemangioma-derived progenitor/stem cells (HemSCs), endothelial progenitor cells (HemEPCs), ECs (HemECs) and perivascular cells (Hem-pericytes).
HemSC
The etiology of IH is still unknown. Over the years, several researchers speculated an aberrant embryonic developmental in situ or placental embolic origin of IH during early fetal life. The ‘placental theory’ is based on the co-expression of placental antigens, such as glucose transporter-1 (GLUT-1), merosin, Lewis Y antigen, FCγRIII, and type 3-iodothyronine deiodinase in IH ([11–13]). In addition, a higher incidence of IH has also been observed following amniocentesis and chorionic villous sampling (CVS) [14, 15]. Supporting the hypothesis of dysregulated embryonic differentiation is the identification of progenitor/stem cells in IH. In 2008 the Bischoff group isolated a primitive mesenchymal cell [16] using anti-CD133-coated magnetic beads. These rare cells, comprising 0.1–1% of the cells in proliferating phase IH have the ability to self-renew and undergo multi-lineage differentiation. Differently from Bone marrow mesenchymal progenitor cells, HemSC differentiate not only towards adipocytes, osteocytes and chondrocytes but also into endothelium. When implanted sub-cutaneously into immune-deficient mice, the HemSC form GLUT1+ vessels. Tracing the cells in vivo demonstrated that HemSC form the two layer of the capillaries- endothelium and pericytes [17]. Later, HemSC differentiate into adipocytes [16]. Other groups reported similar results. Xu et al. isolated stem cells from IH using selective culture media and grew tumor sphere that expressed CD133 and the progenitor cell marker SALL4. Injection into immunodeficient mice produced GLUT1 positive tumors [18]. Itinteang T et al. demonstrated the expression of embryonic stem cell (ESC) markers, Nanog, SALL4, and CD133 in the proliferative phase of IH with reduced expression during lesion involution [19, 20]. Taken together, these findings suggested that IH is not only a disorder of angiogenesis (i.e., the sprouting of new vessels from existing ones) but also a disorder of vasculogenesis (i.e., the de novo formation of new blood vessels from stem cells)
Endothelial Cells
Endothelial cells constitute about 30% of the proliferating tumor. Morphologically the cells are plump in the proliferating tumor and spindle shape in the involuting phase [21]. In vitro studies showed that both in terms of cell morphology and protein expression hemangioma endothelial cells are more characteristic of embryonic microvascular endothelial cells than that of postembryonic cells [8]. The cells express typical endothelial-specific markers such as von Willebrand Factor, CD31/PECAM-1, and E-selectin KDR, TIE-2, and VE-cadherin [22, 23]. In addition, North and colleagues showed that glucose transporter-1 (GLUT1) is expressed on hemangioma endothelium, whereas no expression is noted in other types of vascular tumors and vascular malformations [24]. Consequently GLUT1 became a useful diagnostic marker. HemEC were shown by Boye and colleagues to be clonal, suggesting they arise from a common precursor [22].
Hemangioma endothelial progenitor cells (HemEPC) are less differentiated cells that were isolated based on the human stem cell marker CD133. These cells constitute a small percentage (0.1–2%) of the total endothelial cells. HemEPC, similarly to HemEC, share properties with cord-blood endothelial colony–forming cells (CB-ECFCs) including paradoxical stimulation by endostatin, suggesting that the two cell types have immature phenotype [25, 26].
Myeloid Cells and Macrophages
The presence of myeloid cells in IH, and more specifically macrophages, have been shown in several studies [27, 28]. Macrophages perform phagocytic clearance of dying cells and protect the host through innate immunity, both as resident tissue macrophages and as monocyte-derived recruited cells during inflammation. Classically, macrophages have been divided into two types: The M1-polarised macrophages are activated by microbial antigens and respond to Th1 immune cells by the secretion of pro-inflammatory cytokines. The M2-polarized macrophages secrete anti-inflammatory pro-angiogenic factors in response to Th2 cells [29]. Both M1 and M2 Macrophages were shown to be more prevalent in proliferating IHs than in involuting lesions [27, 30], with M1 being more prevalent. The cells are located in the interstitium, between the vessels, and were shown to increase the proliferation of HemSC. In addition, conditioned media from either M1- or M2-polarized macrophages significantly suppressed the adipogenesis of HemSC [30]. Thus, macrophages may have a role in the proliferation of IH.
Pericytes
Pericytes are contractile mural cells that are located around the EC of capillaries and venules. The cells that can be distinguished from vascular smooth muscle cells by the expression of distinct set molecular markers including platelet-derived growth factor receptor β (PDGFRβ), CD146, aminopeptidases A and N (CD13), endoglin, neuron-glial 2 (NG2) [31–33]. Throughout the vasculature pericytes have a central role in endothelial barrier development and maintenance of integrity [34]. In addition, the microvascular tone is regulate, in large, by the pericytes [35].
In IH, pericytes are abundant in both the proliferating and the involuting phases [20]. In vitro and in vivo studies have shown that HemSC differentiate into pericytes upon direct contact with EC. Jagged-1, a Notch ligand, is required for the differentiation process [17]. Thus, the source of the pericytes in the tumor might be the HemSC. Boscolo et al. demonstrated that IH pericytes differ from normal pericytes both by their phenotype and their expression profile [36]. Hemangioma pericytes have increased proliferation, increased vessel formation in vivo, and decreased ability to suppress proliferation and migration of endothelial cells. In addition, the cells secrete more VEGF-A, a critical cytokine for IH proliferation [36, 37].
Propranolol Treatment
Historically, systemic glucocorticoids were the mainstay therapy for complicated IH, with interferon alfa and vincristine used for unresponsive tumors. However, in recent years, beta-blockers, most specifically propranolol, have become the first line treatment. In 2008, Leaute-Labreze et al. published their serendipitous discovery of the rapid clinical effect of propranolol on IHs. In two children treated with propranolol for cardiopulmonary indications [38] rapid regression of the tumor was observed. Since then, many retrospective studies and case reports [39, 40] and few, placebo-controlled trials [41, 42] have supported the efficacy of this treatment. A meta-analysis of 35 studies comprising 795 patients treated with propranolol showed response rate of 97%, compared to pooled response rate of 69% to steroids after 12 months of follow-up (p < 0.001) [43]. The dosing is most frequently 2 mg/kg of body weight per day and there is still a lack of consensus regarding the protocols for initiation of the drug and ongoing monitoring [44, 45]. Propranolol use is not devoid of side effects. These include diarrhea, sleep disorders, bronchial hyperreactivity, cold hands and feet as well as—rarely—hypoglycemia that may be life threatening [41, 46]. Consequently, a number of beta blockers other than propranolol (e.g. timolol and nadolol and atenolol) showed similar efficacy with potentially fewer adverse side-effects [47, 48].
Beta Adrenergic Signaling
The β-adrenergic signaling pathway mediates fight-or-flight stress responses through the sympathetic nervous system (SNS) [49]. The sympathetic nerve fibers innervate all tissues in the body and secrete the catecholamine neurotransmitter norepinephrine (NE) in response to environmental, psychological or physiological stimuli [50, 51]. Upon activation, catecholamine levels rises both in tissues and in circulating blood via the release of epinephrine (E) from the adrenal medulla chromaffin cells and NE overflow from vascular neuro-muscular junctions.
The biological effects of NE and E are mediated by three receptor families: α1, α2 and β -adrenergic receptors. Ligation of β-receptors by NE and E activates the Gαs guanine nucleotide-binding protein to stimulate adenylyl cyclase synthesis of cyclic 3′–5′ adenosine monophosphate (cAMP). Subsequently, protein kinase A (PKA) is activated and, in turn, phosphorylates serine or threonine residues on myriad of target proteins involved in cells proliferation, differentiation, morphology and motility. Gene expression studies have demonstrated that approximately 20% of human genes are affected by PKA-induced phosphorylation [52, 53]. An additional key effector of cAMP is guanine nucleotide Exchange Protein activated by Adenylyl Cyclase (EPAC). EPAC stimulates the Ras-like guanine triphosphatase Rap1A, which then activates downstream effectors B-Raf, MEK1/2, and ERK1/2A [54]. Propranolol is an orthosteric antagonist of both β1- and β2-adrenergic receptors. In addition, it functions as central serotonin 5-HT receptor antagonist, inhibitor of noradrenaline reuptake and indirect agonist of α-adrenergic receptors [55]. The drug exists as a pair of optical isomers: S(−)propranolol and R(+)propranolol. The enantiomers bind with relatively large differences in affinity to the β-adrenoceptors [55]. Propranolol, as other pharmacological antagonists of the adrenergic receptors, counteract the agonists via the same signaling pathways.
Stress and the β-adrenergic system have been shown to have many effects on tumor biology. Though with some conflicting results, the use of β-blockers has been linked to increased survival of patients with solid cancers [56]. In addition, stressful life conditions have been shown to correlate with less favorable prognosis of cancer patients. In mouse models of tumors β-adrenergic agonists have also been found accelerate tumor progression and metastasis [57, 58]. As expected by the high percentage of cAMP responsive genes, many cellular processes in tumors were shown to be modulated by the β-adrenergic system, including expression of pro-inflammatory cytokines, of macrophages recruitment, angiogenesis, invasiveness and apoptosis [51, 59].
The study of the mechanism of action of propranolol on IH is much “Younger”. Yet, much progress has achieved during recent years. Studies done on human cells isolated from IH in vitro and in vivo, on mouse models, revealed an effect on three major processes that will be detailed below: vascular tone, angiogenesis and vascuogenesis.
Vascular Tone
Following the administration of propranolol, a rapid change in the tumor consistency and color is typically noticed, especially with deep-seated IH [40, 60]. This raises the question of whether propranolol has an effect on the tumor vacular tone via vasoconstriction. Indeed, propranolol has been shown to decrease tissue blood flow to many organs following single administration [61–63]. Particularly in the skin, adrenaline-induced vasoconstriction has been shown to be increased by oral propranolol [64]. However, additional mechanisms might be involved in the slower, long-term effect of propranolol. A potential target of this mechanism of action is the pericyte. Pericytes are regulators of microvascular tone. Bosclo et al. demonstrated that pericytes from proliferating IH have lower density of the cytoskeleton component F-actin fiber compared to involuting Hemangioma pericytes and retinal pericytes. Also, these cells exhibit lower contractile capacity compared to normal pericytes [36]. In another work from the same group Lee et al. demonstrated that Epinephrine-induced relaxation of IH pericytes was prevented by propranolol. Using siRNA assay it was demonstrated that both the relaxation and its prevention by propranolol were mediated by the β2 receptor [65]. Interestingly, cultured pericytes from other sources have been shown to constrict, not relax, in response to catecholamines [66, 67]. Thus, the response of pericytes to NE and its blockers might be cell and context dependent.
In addition to direct effect of propranolol on the pericytes to induce contraction, it might exert its effect indirectly via the blocking of Nitric oxide release from HemEC. The endothelial isoform of nitric-oxide synthase (eNOS), is a key determinant of vascular tone. [68, 69] In different tissues and experimental systems, diverse adrenergic receptors subtypes have been shown to modulate of eNOS expression and activity, including the β1, 2 and 3 adrenergic receptors [70, 71], eNOS protein expression has been demonstrated to be significantly decreased in involuting versus proliferating hemangiomas [72]. In patients treated with propranolol, a significant decrease in the expression of eNOS in hemangioma tissues was noted compared with the age-matched untreated controls [72]. In addition, Wei-li Yuan have shown that serum concentrations of eNOS declined gradually during the first 2 months of propranolol treatment for IH [73].
Angiogenesis
Vascular endothelial growth factor (VEGF) has been shown to play a pivotal role in the angiogenic process in general and specifically in IH proliferation. The VEGF family consists of five ligands VEGF-A, VEGF-B, VEGF-C, VEGF-D and placental growth factor (PIGF). These ligands bind to three main subtypes of VEGF receptors: VEGFR-1 (Flt-1), VEGFR-2 (KDR/Flk-1)and VEGFR-3 [74]. Several works showed over-expression of VEGF-A in proliferating hemangioma tissue and in serum of patients with IH, compared to healthy controls [37, 75–77]. Moreover, we have shown that silencing the expression of VEGF-A in HemSC by short hairpin RNA (shRNA) was sufficient to block blood vessel formation in vivo [37]. NE enhances VEGF-A expression of both normal and tumoral cell types, [78–80]. Propranolol has been shown to reverse this effect via the blocking β1 and β2 adrenergic receptors [78–81]. Several lines of evidence suggest that propranolol acts at least in part through the blocking of VEGF. First, serum VEGF levels were shown to decrease noticeably in 91% of patients after a single month of propranolol treatment [82]. Similar results were reported by another group following the administration of relatively low doses of propranolol [73]. Second, in vitro studies have demonstrated that the NE agonist isoprenaline increased the expression of VEGF-A and the phosphorylation of VEGFR-2 in HemECs in a β-adrenergic receptor- and extracellular-signal-regulated kinase (ERK) -dependent manner. This response was blocked by β-adrenergic blockers. In HemSC, Ling Zhang et al. showed that propranolol at physiological concentrations leads to dose-dependent suppression of VEGF expression, at the mRNA and the protein level [83].
An additional major regulator of angiogenesis is Hypoxia-inducible factor (HIF)-1α. During hypoxia, HIF-1α binds the regulatory region of the VEGF gene, inducing its transcription and initiating its expression [84, 85]. HIF-1α expression has shown to be increased in the endothelium of proliferating hemangioma compared with involuting tissues [77]. Recently, it has been shown that HIF-1α was upregulated in the serum, urine and tumor tissues of IH, and treatment of propranolol markedly inhibited its expression. In vitro, in HemEC, overexpression of HIF-1α blocked the inhibitory effects of propranolol on VEGF expression [86].
Several groups have demonstrated a direct pro-apoptotic or anti proliferative effect of propranolol on HemEC. However, a major concern is the high concentrations of propranolol required for these effects. These drug levels are unlikely to be present in the tumor’s microenvironment [87]. It is possible, though, that propranolol works by opposing the growth promoting effects of catecholamines. Ji et al. showed that HemECs proliferation increased in response to isoprenaline via regulation of thee cell-cycle proteins cyclin D1 and its associated kinases, CDK-4 and CDK-6. These effects were reversed by β-adrenergic receptor antagonists. Of note, the antagonists had no effect on basal cell proliferation, but significantly decreased ISO-induced cell proliferation and cell viability [88]
Vasculogenesis
Vasculogenesis, the creation of blood vessels de-novo from stem/progenitor cells, contributes the proliferation of IH. Thus, it is appealing to hypothesize that propranolol acts as an inhibitor of this process, as was shown for corticosteroids and rapamycin [89]. Against this hypothesis is the fact that late regrowth of the hemangioma seen in a subset of patients after cessation of the treatment [90]. This might mean that propranolol does not target the HemSC or even prevents their terminal differentiation or apoptosis. Effect of the adrenergic system on non-hemangioma stem/mesenchymal cells has been shown by several works. For example, NE has been shown to induce brown adipocyte differentiation of mesenchymal progenitors within white adipose tissue [91]. Also, an effect of NE on mesenchymal stem cells adipogenesis has been demonstrated in-vitro cell [92]. In work done on HemSC, Wong et al. reported that Propranolol-treated cells had a more robust response to adipogenic induction when compared to vehicle-treated HemSCs [93]. In a follow-up work the same group has shown that this adipogenic differentiation is characterized by improper adipogenic gene expression [94]. Similar results were reported by another group [95]. However, these results were achieved in very high, non-physiological concentration of propranolol.
Concluding Remarks
Although benign, IH commonly leads to disfigurement and negatively affects the life quality of patients and their families. The discovery of beta blockers have revolutionized the field and offered effective treatment for these lesions. However, not all tumors respond and their use is not without risk. Understanding the pathogenesis of IH and the mechanisms of action of beta blockers could potentially lead to novel pharmacological approaches which are safer and more effective. Advances have been made in recent years, mainly through the isolation and characterization of the cellular components of IH and the creation of relevant animal models. However, due to the complexity of the adrenergic system, more studies are needed in order to establish the relevance of each signaling pathway and cellular effect.
References
1.
2.
3.
Enjolras O, Mulliken JB. The current management of vascular birthmarks. Pediatr Dermatol. 1993;10(4):311–3.CrossRefPubMed
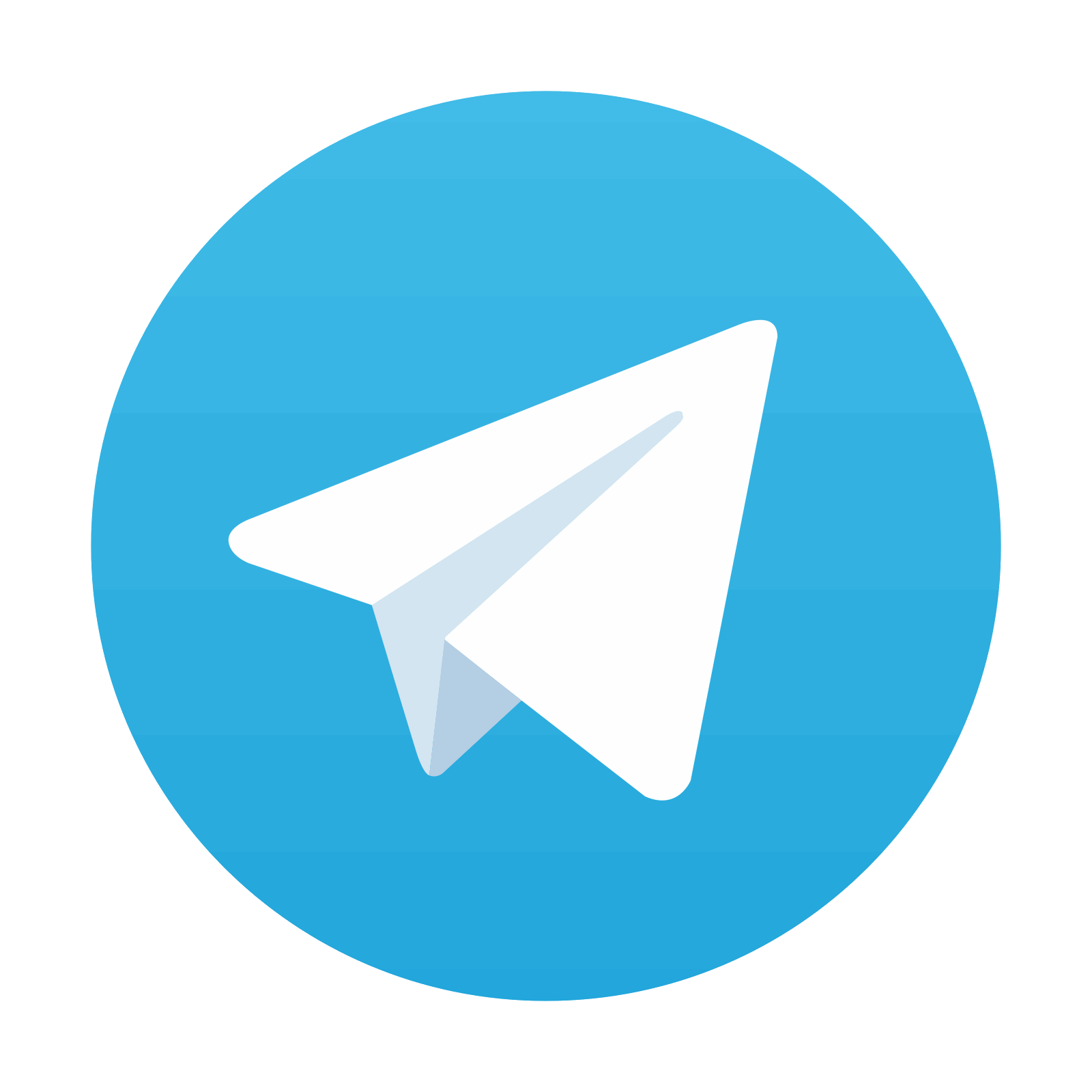
Stay updated, free articles. Join our Telegram channel

Full access? Get Clinical Tree
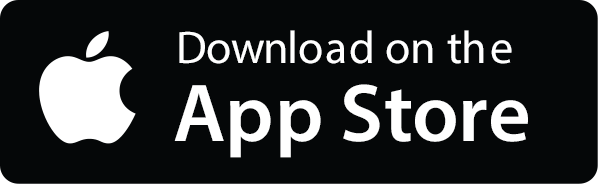
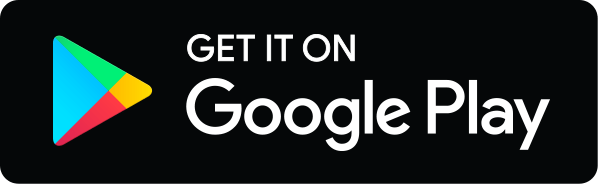
