Fig. 20.1
Schematic representation of radical polymerization. Hydrogels are formed by the copolymerization of HEMA with EGDMA using AIBN as the radical initiator

Fig. 20.2
Preparation of PVA hydrogels cross-linked by acyl dichlorides

Fig. 20.3
Schematic representation of the radiation method to design hydrogels
Physical cross-linking of hydrogels also avoids the use of chemical cross-linking agents. Such agents can potentially inactivate the active principle and covalently link it to the hydrogel network. Examples of ionically cross-linked alginate hydrogels have been reported (Grant et al. 1973). Alginate is a family of linear polysaccharides composed of mannuronic acid (MA) and guluronic acid (GA). The chemical composition and sequence of MA and GA residues depend on the source from which the alginate has been extracted. The gelation of alginate is mainly achieved by the exchange of sodium ions with divalent cations such as Ca2+, Cu2+, Zn2+, or Mn2+, which can form cation bridges between adjacent molecules. The “egg-box” model of Grant et al. (1973) is generally taken into consideration to explain the formation of a rodlike cross-linked complex due to the bounding of the divalent cations in the interchain cavities. Some polymeric complexes can be held together by hydrogen bonds: poly(acrylic acid) and poly(methacrylic acid) provide physically cross-linked hydrogels with poly(ethylene glycol) due to the formation of hydrogen bonds between the oxygen of poly(ethylene glycol) and the carboxylic groups of the acrylic polymers (Eagland et al. 1994). Another physical method for producing physically cross-linked hydrogels is the formation of crystalline regions in the polymer network, obtained by casting dilute, aqueous solutions of poly(vinyl alcohol), then cooling to −20 °C, and thawing back to room temperature several times (Stauffer and Peppas 1992). These freeze/thawed gels have demonstrated enhanced physical properties, such as high mechanical strength and high elasticity, that make them suitable for biomedical applications.
Finally, physically cross-linked hydrogels can be obtained by hydrophobic modification of polymers and in particular of polysaccharides such as chitosan, dextran, pullulan, and carboxymethyl curdlan (Noble et al. 1999; Sludden et al. 2000; Cerchiara et al. 2002). Glycol chitosan substituted with palmitoyl chains is an example of a hydrophobized polysaccharide. The attachment of hydrophobic groups to glycol chitosan yields an amphiphilic polymer capable of self-assembly into vesicles (Uchegbu et al. 1998). Non-covalent cross-linking is achieved by the hydrophobic interactions of the palmitoyl groups and a gel matrix is formed. Finally, our research group (Cerchiara et al. 2002) reported physically cross-linked chitosan hydrogels with lauric, myristic, palmitic, or stearic acid prepared by freeze drying and studied for transdermal use (Fig. 20.4). These polymers produce hydrogels with different functional properties related to the different acyl chains introduced in the polymer structure. In particular, the permeation of hydrophilic substances through the skin can be modulated by increased or decreased drug solubility due to the interaction of the different acyl chains with the stratum corneum.


Fig. 20.4
Structural representation of physically cross-linked chitosan hydrogels
20.2 Applications of Hydrogels in Transdermal Drug Delivery
Transdermal delivery is an attractive and promising alternative compared to conventional administration routes (e.g., oral and injectable) for transport through the skin into the blood circulation of drugs such as hydrophobic small molecules, hydrophilic molecules, and macromolecules. In fact, this route offers several advantages over conventional routes (Peppas et al. 2000; Brown et al. 2006; Prausnitz and Langer 2008):
Is noninvasive and pain-free, with no trauma or risk of infection (Denet et al. 2004), thus resulting in an enhanced patient compliance
Maintains constant drug levels in the blood, eliminating plasma peaks and valleys associated to oral and injectable administration
Avoids gastrointestinal tract and circumvents hepatic first-pass metabolism, thus resulting in a lower drug amount administered and in a reduction of systemic side effects
Is inexpensive
In spite of the advantages, major disadvantage of transdermal drug delivery is that the drug itself or the materials used to fabricate the vehicles may sometimes induce an irritation or sensitization reaction of the skin (Kurihara-Bergstrom et al. 1991; Murphy and Carmichael 2000; Ale et al. 2009; Wohlrab et al. 2011). Moreover, few molecules have been successfully delivered transdermally, mainly due to the stratum corneum that forms a barrier to the permeation of hydrophilic drugs, especially macromolecules such as proteins, peptides, and vaccines.
Consequently, research trends are focusing on approaches to overcome the barrier presented by the skin, including physical penetration enhancers such as microneedles (Henry et al. 1998), iontophoresis and electroosmosis (Pikal 2001), electroporation (Hu et al. 2000), radiofrequency energy (Sintov et al. 2003), and chemical penetration enhancers such as sulfoxides, alkanones, alcohols, polyols, amides, fatty acids, fatty acid esters, surfactants, terpenes, organic acids, and cyclodextrins (Thong et al. 2007). Recent studies have introduced a new category of transdermal penetration enhancers such as positively charged polymers. In fact, Taveira et al. (2009) described that chitosan interacts with negative charges in the skin improving drug penetration in the deeper layers, while He et al. (2008, 2009) demonstrated that chitosan and its derivatives such as N-trimethyl chitosan and mono-N-carboxymethyl chitosan are able to change the secondary structure of keratin in stratum corneum leading to a less organized structure of this protein and enhancing transdermal permeation of drugs.
As regards the transdermal drug delivery systems, the traditional formulations as plasters, cream, and ointments have been replaced in order to minimize skin irritation, promote adhesion properties, guarantee dosage flexibility, enhance patient acceptability, and improve ease of use. In this context, particular attention has been paid to the formulations based on hydrogels such as semisolid systems and film-based systems (matrix-type systems, membrane-coated systems, film-forming solution).
20.2.1 Semisolid Vehicles
Hydrogels are three-dimensional networks based on linear hydrophilic polymers that are able to absorb large amounts of water, remaining insoluble due to the presence of chemical or physical cross-links (Peppas et al. 2000). The relatively high water content makes them a good alternative to other dosage forms such as creams, ointments, and patches, enhancing skin moisturization and elasticity and providing a better feel when applied to the skin. The most important and well-known polymers used for preparing these hydrogels are biopolymers such as polysaccharides (starch, cellulose, chitin, alginate, hyaluronate) or proteins (collagens, gelatins, caseins, albumins) and synthetic polymers such as polyvinyl alcohol, polyvinylpyrrolidone, polyethylene glycol, and polyacrylates.
Chitosan, a polysaccharide comprising copolymer of glucosamine and N-acetylglucosamine, derived by the partial deacetylation of chitin, is a nontoxic and bioabsorbable polymer (Muzzarelli et al. 1988; Luppi et al. 2010a) extensively studied for the release of many drugs. Our research group (Cerchiara et al. 2002) described physically cross-linked chitosan hydrogels with lauric, myristic, palmitic, or stearic acid able to enhance the skin permeation of propranolol hydrochloride selected as a hydrophilic model drug. The aim of the work was to improve the permeation of drugs through biological membranes, using hydrogels made of amphiphilic polymer (Noble et al. 1999). The concomitant presence of hydrophobic and hydrophilic groups in the polymer influenced the swelling properties. So, at pH 7.4 all hydrogels swelled slowly and their behavior influenced the drug release. Among the different chitosan gels, chitosan laurate and chitosan myristate enhanced drug permeation through the skin with respect to chitosan palmitate and chitosan stearate hydrogels (flux values (mg/h cm2) of propranolol hydrochloride from physically cross-linked chitosans oversaturated hydrogels through porcine skin were 1.00 ± 0.02 for chitosan laurate, 0.87 ± 0.05 for chitosan myristate, 0.47 ± 0.03 for chitosan palmitate, and 0.37 ± 0.01 for chitosan stearate). This could be explained by the interaction of the hydrogels with the stratum corneum, increasing the solubility of the drug in the skin.
Another example of hydrophilic and biocompatible polymer used to design hydrogels able to release hydrophilic drugs through the skin is polyvinyl alcohol. Polyvinyl alcohol cross-linked with succinyl, adipoyl, or sebacoyl chloride was employed as a supporting material to release propranolol hydrochloride. In particular, these hydrogels increased the transdermal permeation of drug, and as described in the previous work (Cerchiara et al. 2002), this effect seems to be linked to an increased drug solubility in the skin, probably produced by the interaction of the polymer with the stratum corneum. Moreover, the maximum enhancement of the drug permeation has been observed in the presence of the higher degree of cross-linking and the shorter length of the cross-linker acyl chain (Orienti et al. 2000).
Luppi and coworkers (2003) used also the cross-linked poly(methyl vinyl ether-co-maleic anhydride) (GZ) as a topical vehicles for pyridoxine hydrochloride, selected as a hydrophilic model drug. In particular, poly(methyl vinyl ether-co-maleic anhydride) was cross-linked with ethylene glycol (GZ-ET), butanediol (GZ-BUT), 1,6-exandiol (GZ-EX), 1,8-octanediol (GZ-OCT), 1,10-decanediol (GZ-DEC), or 1,12-dodecanediol (GZ-DOD). In vitro permeation studies were influenced by the nature of the cross-linker: the decrease in cross-linker acyl chain length provides vehicles accelerating drug permeation through the skin. In fact, flux values (mg/h cm2) of pyridoxine hydrochloride from hydrogels through porcine skin were 1.29 ± 0.12 for GZ, 5.83 ± 0.22 for GZ-ET, 4.91 ± 0.14 for GZ-BUT, 4.32 ± 0.10 GZ-EX, 3.82 ± 0.09 GZ-OCT, 3.25 ± 0.11 GZ-DEC, and 2.42 ± 0.12 GZ-DOD.
20.2.2 Film-Based Vehicles
Film-based vehicles are the widest utilized and studied transdermal delivery systems based on hydrogels. They are generally distinguished in matrix-type systems, membrane-coated systems, and film-forming solution.
The matrix-type systems are essentially a polymeric layer containing the drug, eventually added with an adhesive layer to enhance the bioadhesion and an impermeable layer to force the release to the skin. In absence of the adhesive layer and in order to assure a controlled delivery of the drug, it is necessary to choose polymers able to guarantee an intimate and prolonged contact with the skin after the application and flexibility and elasticity sufficient to follow the movements of the skin. An adhesive hydrogel patch based on a hydrophilic matrix of poly(N-vinylpyrrolidone) and oligomeric short-chain poly(ethylene glycol) was reported by Feldstein and coworkers (1996). They observed that the delivery rates of drugs with various chemical structures (propranolol, glyceryl trinitrate, isosorbide dinitrate) from the hydrophilic transdermal systems were higher than from the hydrophobic ones (stirene-butadiene rubber/mineral oil, polydimethylsiloxane/ silicone oil, polyisobutylene/mineral oil), and the drug delivery from the hydrophilic matrix across human cadaver skin epidermis or skin-imitating Carbosil membrane in vitro was characterized by zero-order drug delivery kinetics up to the point of 75–85 % drug release from initial contents in matrix. Drug delivery rates from the hydrophilic matrix were controlled by the skin or skin-imitating membrane permeability and may be described by Fick’s law (Iordanskii et al. 2000).
Ethylene-vinyl acetate (EVA) matrix was tested as a system for transdermal delivery of atenolol in the presence of plasticizers able to increase the rate of drug release. The effects of drug concentration, temperature, and plasticizers on drug release were investigated. The release rate from EVA matrix was enhanced increasing temperature and drug concentration. In particular, the release rate of drug increased about 1.72-fold when the temperature of release system was raised from 32 to 42 °C and about 1.24-fold when the drug loading dose was increased from 0.5 to 1.5 %. Moreover, drug release from the polymeric matrix followed a diffusion-controlled model, where the quantity released per unit area was proportional to the square root of time. Among the plasticizers used such as alkyl citrates and phthalates, tributyl citrate showed the best enhancing effects: flux values (μg/cm2/h1/2) were 8.056 and 5.327 for EVA matrix containing tributyl citrate and EVA matrix without plasticizer, respectively. The results obtained confirmed that ethylene-vinyl acetate matrix could be used for transdermal delivery of hydrophilic drug (Kim and Shin 2004).
Padula and coworkers (2003) proposed a polyvinyl alcohol film not adhesive in the dry state, but bioadhesive when applied on wet skin. The film was applied to the skin in the presence of a certain amount of water. Water swelled the film on the surface in contact with the skin, transforming a dry polymeric matrix into a jellified polymer layer. This particular film is flexible, is mechanically resistant, and can avoid skin occlusion because of its permeability to water vapor. Compared to a typical patch, the bioadhesive film has a monolayer structure which includes backing, adhesive, and drug reservoir functions. This simple delivery system composed of a smaller number of layers simplifies the preparation procedure of transdermal patches and represents a great innovation in the field of transdermal patch.
Another polymer that represents a good candidate as a starting material for film-based vehicles because of its good film-forming properties is chitosan (Mengatto et al. 2012). Frequently, chitosan is modified by cross-linking reactions to achieve films with improved mechanical properties and obtain an efficient control of drug delivery. In this context, films based on polyelectrolyte complexes were developed for topical and transdermal administration of drugs by Silva et al. (2008). The complexes were prepared with chitosan and different polyacrylic acid polymers, cross-linked with allyl pentaerythritol (Carbopol 71G NF®) or divinylglycol (Noveon AA-1®) at different cross-linking densities. The interaction between the polymers was maximized controlling the preparative conditions of complexes, and the film properties were improved by means of different plasticizers (glycerol or polyethylene glycol 200), a moisturizing agent (Hydrovance®), and an hydrophilic pressure sensitive adhesive (polyvinylpyrrolidone/polyethylene glycol 400). Between the different vehicles obtained, the film prepared by cross-linking with Noveon, plasticized with glycerol and covered with adhesive, has shown very good flexibility, resistance, and bioadhesion, making it a good candidate for further incorporation of drugs for topical and transdermal administration. Recently, chitosan-polyvinyl alcohol blend reticulated with glutaraldehyde has been utilized to prepare transdermal film suitable for insulin release in diabetes chemotherapy (Zu et al. 2012). The hydrogel obtained had a honeycomb-like structure and showed good mechanical and thermal properties. Moreover, the in vitro release studies showed that insulin release is comply with Fick’s first law of diffusion showing a high permeation rate (4.421 μg/(cm2h)).
Transdermal delivery represents also an important opportunity for vaccine administration (Prausnitz and Langer 2008). In fact, although vaccines are generally large entities such as macromolecules or viral particles, their small dose facilitates transdermal administration. Ishii et al. (2008) describes simple, easy-to-use, noninvasive transcutaneous vaccination system, formed by an adhesive matrix. This patch is composed of cross-linked acrylic medical adhesive, octyldodecyl lactate, glycerin, and sodium hyaluronate and delivers antigenic proteins to Langerhans cells resident in the epidermal layer without destroying or removing the stratum corneum and induces Th2 (Type 2 helper T cells)-dominant immune response (production of neutralizing immunoglobulin G1 antibodies), effectively preventing viral and bacterial infection.
Finally, Luppi et al. (2010 b) formulated transdermal hydroxypropyl methylcellulose-based films containing chlorpromazine hydrochloride for the treatment of psychotic disorders. Film composition was modified by incorporating a chemical permeation enhancer or binary enhancer combinations (oleic acid or polysorbate 80, or both) and a plasticizer (propylene glycol). Both oleic acid and polysorbate 80 had significant effect on drug permeation with respect to the control formulation and films containing a mixture of oleic acid and polysorbate 80 provided the best enhancement activity for chlorpromazine. In fact, the amount of chlorpromazine hydrochloride permeated through pig ear skin from hydroxypropyl methylcellulose films after 100 h were 15.2 ± 0.7 mg/cm2 for film containing oleic acid (2.15, % w/w on dry basis) and polysorbate 80 (2.15, % w/w on dry basis) and 6.5 ± 0.3 mg/cm2 for control formulation (film with the same composition, but without permeation enhancers). Moreover, also the hydroxypropyl methylcellulose type and the different concentration of drug and plasticizer contributed to modulate drug permeation. A decrease of hydroxypropyl methylcellulose viscosity, as a function of its molecular weight, and an increase in propylene glycol and chlorpromazine content provided higher cumulative amounts of drug permeated. These results confirm that chlorpromazine permeation can be easily modulated by varying the composition of hydroxypropyl methylcellulose-based films.
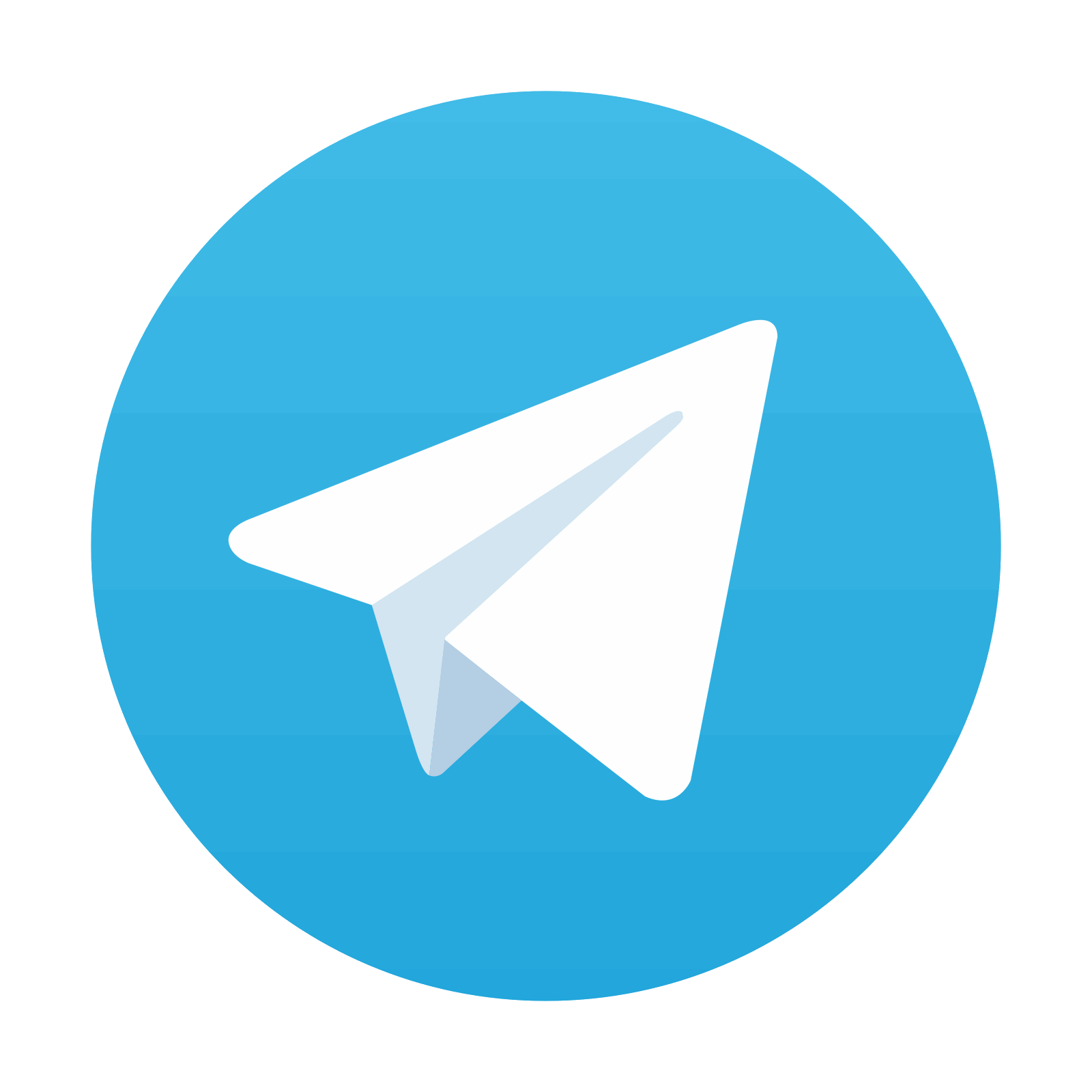
Stay updated, free articles. Join our Telegram channel

Full access? Get Clinical Tree
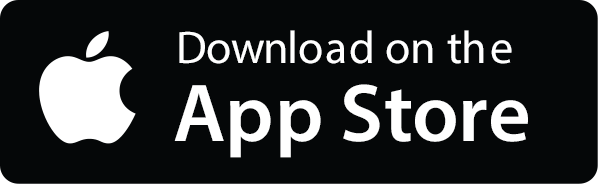
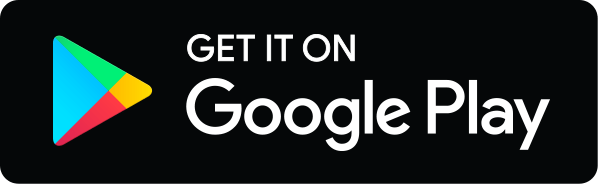