Category
Time of symptoms after HCT or DLI
Presence of acute GVHD features
Presence of chronic GVHD features
Acute GVHD
Classic acute GVHD
≤100 d
Yes
No
Persistent, recurrent, or late-onset acute GVHD
>100 d
Yes
No
Chronic GVHD
Classic chronic GVHD
No time limit
No
Yes
Overlap syndrome
No time limit
Yes
Yes
The clinical manifestations of acute GVHD occur in the skin, gastrointestinal tract, and liver. Total bilirubin levels, quantification of diarrhea volume, and extent and type of skin involvement are used to stage the disease ([23, 29, 53, 89], Table 27.2). Hepatic involvement is characterized by elevated total bilirubin. Gastrointestinal tract involvement presents as anorexia, abdominal pain, nausea/vomiting, and secretory diarrhea.
Table 27.2
Staging and grading of acute GVHD
Stage | Skin | Liver | Gut |
---|---|---|---|
1 | Rash <25 % BSA | Bilirubin 2 mg/dL to <3 mg/dL | Diarrhea 500–1,000 mL/day or persistent nausea |
2 | Rash 25–50% BSA | Bilirubin 3–6 mg/dL | Diarrhea 1,000–1,500 mL/day |
3 | Rash >50% BSA | Bilirubin 6–15 mg/dL | Diarrhea >1,500 mL/day |
4 | Erythroderma w/ bullae formation | Bilirubin >15 mg/dL | Severe abdominal pain with or without ileus |
Grade | |||
I | Stages 1–2 | None | None |
II | Stage 3 | Stage 1 | Stage 1 |
III | Stages 2–3 | Stages 2–4 | |
IV | Stage 4 | Stage 4 |
Skin is most commonly affected and is usually the first organ involved, often coinciding with engraftment of donor cells [28, 29]. Skin manifestations range from a mild, asymptomatic exanthema-like eruption to full-thickness skin loss resembling toxic epidermal necrolysis . Acute GVHD initially presents with erythematous-dusky macules and papules of the volar and plantar surfaces and ears that may rapidly become a diffuse morbilliform exanthema [23]. Very early involvement may manifest as erythema limited to hair follicles. Erythroderma may develop, and, in severe cases, spontaneous bullae with skin sloughing resembling toxic epidermal necrolysis may occur. Pruritus is variable and is not useful to distinguish acute GVHD from other causes of the rash. Mucous membranes can also become involved [23].
27.2.2 Chronic GVHD
Chronic GVHD is a multisystem disorder that may affect nearly any organ and is now known to have features resembling autoimmune and other immunologic disorders. The most common organs involved are the skin followed by oral mucosa, liver, and eyes [23, 53]. Risk factors for chronic GVHD include older recipient age, history of acute GVHD, the use of female donor for male recipient, use of DLI, use of unrelated or HLA-mismatched donors, and more recently, the use of growth-factor–mobilized peripheral blood leukocytes as opposed to marrow as a source of stem cells [12, 32]. As mentioned earlier, any manifestation of GVHD that was present (or continued) at 100 days after HCT or thereafter was arbitrarily defined as chronic GVHD even if the clinical manifestation was indistinguishable from that of acute GVHD. The current consensus is that acute or chronic disease definition is based primarily on clinical manifestations and histologic findings [32].
Chronic GVHD of the skin is remarkably variable in clinical presentation. In the past, an early lichenoid stage and a late sclerodermoid stage could be distinguished, however, in appreciation of the tremendous variability in clinical presentation, it is no longer useful to dichotomize chronic GVHD of the skin into either “lichenoid” or “sclerodermoid” categories [23]. Sclerotic or nonsclerotic (used to be lichenoid) are the terms that are now used [53]. Nonsclerotic cutaneous GVHD is characterized by epidermal changes that resemble lichen planus , keratosis pilaris, ichthyosis, poikiloderma , papulosquamous/psoriasiform/eczematous rash, dyspigmentation, or acral erythema. Sclerotic changes may resemble lichen sclerosus , morphea , systemic sclerosis , or eosinophilic fasciitis [23, 53]. In contrast to systemic sclerosis, sclerotic involvement of the face, fingers, and toes (sclerodactyly) and Raynaud phenomenon is less common.
New consensus criteria for the diagnosis and staging of chronic GVHD have recently been developed by the National Institutes of Health Consensus Development Project [32] in which definitions of skin involvement as well as other organs have been proposed. According to these criteria, the following skin manifestations are diagnostic of chronic GVHD and therefore do not require a biopsy: poikiloderma, lichen planus-like eruptions, lichen sclerosus-like lesions, morphea-like sclerosis, and deep sclerosis/fasciitis. Various other clinical presentations are considered suggestive or distinctive, but are not sufficient to establish the diagnosis of chronic GVHD in the absence of a confirmatory biopsy or other organ manifestation. For instance, depigmentation is a distinctive feature for chronic GVHD and not seen in acute GVHD but is not sufficiently unique to be considered diagnostic of chronic GVHD [23, 32, 53]. A portion of the NIH criteria describing skin manifestations of chronic GVHD is shown in Table 27.3.
Table 27.3
Signs and symptoms of chronic GVHD
Organ or site | Diagnostic (Sufficient to establish the diagnosis of chronic GVHD) | Distinctive (Seen in chronic GVHD, but insufficient alone to establish a diagnosis of chronic GVHD) | Other features | Common (Seen with both acute and chronic GVHD) |
---|---|---|---|---|
Skin | Poikiloderma | Depigmentation | Sweat impairment | Erythema |
Lichen planus-like features | Ichthyosis | Maculopapular rash | ||
Sclerotic features | Keratosis pilaris | Pruritus | ||
Morphea-like features | Hypopigmentation | |||
Lichen sclerosus-like features | Hyperpigmentation | |||
Nails | Dystrophy | |||
Longitudinal ridging, splitting, or brittle features | ||||
Onycholysis | ||||
Pterygium unguis | ||||
Nail loss (usually symmetric; affects most nails) | ||||
Scalp and body hair | New onset of scarring or nonscarring scalp alopecia (after recovery from chemoradiotherapy) | Thinning scalp hair, typically patchy, coarse, or dull (not explained by endocrine or other causes) | ||
Scaling, papulosquamous lesions | Premature gray hair | |||
Mouth | Lichen-type features | Xerostomia | Gingivitis | |
Hyperkeratotic plaques | Mucocele | Mucositis | ||
Restriction of mouth opening from sclerosis | Mucosal atrophy | Erythema | ||
Pseudomembranes | Pain | |||
Ulcers |
27.3 Histopathology
27.3.1 Acute GVHD
Early changes of GVHD consist of focal basal vacuolation and sparse superficial perivascular lymphocytic infiltrates with exocytosis of individual cells into the epidermis and follicular epithelium [113]. A histological grading system is well established for acute GVHD ([51, 63], Table 27.4). The hallmark feature of acute GVHD is the presence of necrotic keratinocytes accompanied by a sparse dermal lymphocytic infiltrate and basal vacuolar degeneration. In grade 1 disease, there is focal or diffuse vacuolization of the basal layer. In grade 2 lesions, spongiosis and dyskeratotic keratinocytes are identified, with some accompanied by two or more epidermal lymphocytes, a phenomenon known as satellite cell necrosis . The necrotic keratinocytes contain a pyknotic nucleus and eosinophilic cytoplasm. Grade 3 lesions are characterized by subepidermal cleft formation. In grade 4, there is complete separation of epidermis from dermis that correlates with clinical findings resembling toxic epidermal necrolysis. Early GVHD involvement with follicular papules correlates with degenerative changes limited to the hair follicle [34]. The presence of scattered eosinophils does not help differentiate between drug eruptions and GVHD [68]. A recent study demonstrated that a very high number of eosinophils (> average 16 eosinophils/10 high power fields) was necessary to rule out acute GVHD completely [110].
Table 27.4
Histologic grading of acute GVHD
Grade | Histopathologic features |
---|---|
0 | Normal skin or cutaneous disease unrelated to GVHD |
1 | Basal vacuolization of the dermal–epidermal junction |
2 | Basal vacuolization, necrotic epidermal cells, lymphocytes in the dermis and/or epidermis |
3 | Grade 2 plus subepidermal cleft formation |
4 | Grade 2 plus separation of epidermis from dermis |
27.3.2 Chronic GVHD
27.3.2.1 Nonsclerotic Manifestations
As in the case of acute GHVD, chronic cutaneous GVHD exhibits an interface dermatitis, lymphocyte satellitosis, and vacuolar changes at the basal cell layer. Apoptosis within the basilar or lower spinosum layers has been proposed to be the minimal histologic criteria for active GVHD. Acanthosis and wedge-shaped hypergranulosis resembling lichen planus may be seen. In many cases it may not be possible to distinguish acute and chronic epidermal involvement histologically [53, 98].
27.3.2.2 Sclerotic Manifestations
Sclerotic involvement of the papillary dermis may resemble lichen sclerosus with atrophy, hyperkeratosis, follicular plugging, and a pale homogenized appearance of the papillary dermis collagen. If epidermal changes of GVHD are not present, dermal fibrosis with thickened collagen bundles and loss of periadnexal fat involvement may be indistinguishable from idiopathic morphea/scleroderma. Subcutaneous and fascial involvement accordingly reveal changes in the fat septae and fascia, including thickening, edema, and fibrosis, with variable infiltration of lymphocytes, histiocytes, and eosinophils [53, 95, 98].
27.4 Pathophysiology
27.4.1 Acute GVHD
To understand the pathophysiology of acute GVHD, two principles need to be considered. First, acute GVHD reflects exaggerated but normal inflammatory mechanisms mediated by donor lymphocytes infused into the recipient where they function appropriately, given the foreign environment in which they find themselves. Second, the recipient tissues that stimulate donor lymphocytes have often been damaged by underlying disease, prior infection, and the transplant conditioning regimen [28]. As a result, these damaged tissues produce “danger” signals [70] including pro-inflammatory cytokines, chemokines, and increased expression of adhesion molecules, MHC antigens, and costimulatory molecules on host APC s that promote the activation and proliferation of donor immune cells [43, 44, 115]. Animal models have been instrumental in understanding the pathophysiology of acute GVHD and based largely on these experimental models, the development of acute GVHD can be conceptualized in a three-phase process: (1) tissue damage to the recipient by the radiation/chemotherapy pretransplant conditioning regimen and activation of the APCs; (2) donor T-cell activation and clonal expansion; and (3) target tissue destruction [29, 31].
27.4.1.1 Phase I: Conditioning Phase
The first step of acute GVHD begins before donor cells are infused. Tissue damage from the recipient’s underlying disease, infection, and/or the conditioning chemotherapy/radiotherapy leads to inflammatory responses through inflammatory cytokine production and host APC activation. Inflammatory cytokines (TNF-α and IL-1β) have been implicated in the pathogenesis of acute GVHD [43, 48, 49, 88, 115]. Total body irradiation (TBI) is particularly important because it activates host tissues to secrete inflammatory cytokines [43, 115] and induces damage to the gastrointestinal (GI) tract [43, 44]. Increasing evidence suggests that damage to the GI tract during acute GVHD plays a major pathophysiologic role in the amplification of systemic disease. It increases the translocation of inflammatory stimuli such as microbial products including LPS .
Clinical studies first suggested that a correlation between GVHD severity and radiation dose exists [18, 81] and that more severe GVHD occurs after conditioning regimens including radiation therapy than when only chemotherapy is used [19]. Large doses of TBI increase GVHD severity by amplifying the dysregulation of inflammatory cytokines [43]. TBI and allogeneic immune cells synergize to damage the GI tract, thereby permitting increased translocation of LPS into the systemic circulation. This damage, together with increased production of TNF-α by host cells after TBI that promotes further inflammation and additional GI tract damage [44] leads to an increase in the morbidity and mortality in patients with GVHD The GI tract is therefore critical to the propagation of the “cytokine storm ” characteristic of acute GVHD.
LPS is a potent stimulator of inflammatory cytokine production, such as TNF-α and IL-1 , which are important mediators of clinical [1, 48] and experimental GVHD [43, 45, 115]. The production of TNF-α by monocytes and macrophages is transduced by two signals. The first is a priming signal that may be provided by IFN-γ [82] and radiation [43]. The second is a triggering signal provided by bacterial products such as LPS [82]. Translocation of LPS across a damaged gut mucosa provides access to the systemic circulation where LPS triggers monocytes and macrophages primed by the effects of IFN-γ to release cytopathic amounts of inflammatory cytokines [82]. Together with natural killer (NK) and T cells, these cytokines mediate GHVD target organ damage. GVHD is associated with elevated serum levels of both TNF-α and LPS on day 7 after BMT [43]. The importance of LPS in GVHD has further been supported by a mouse study using strains that differ in their sensitivity to LPS as donors in an experimental BM transplant (BMT) system showing that donor resistance to LPS reduces the development of acute GVHD [22]. In murine GVHD, it has been demonstrated that the administration of anti-TNF-α reduces cutaneous and intestinal lesions and mortality [88]. Increased levels of TNF-α have also been found in the serum of allogeneic bone marrow transplant recipients and have been correlated with the severity of GVHD [49]. Administration of recombinant human IL-1 receptor antagonist has been shown to improve acute GVHD and the response to therapy was associated with a reduction of TNF-α mRNA levels in blood mononuclear cells [1].
The Role of APC
The activation of host APCs by inflammatory cytokines that are induced by the underlying disease and the HCT conditioning regimen is thought to be the first step of acute GVHD [29, 31]. A benchmark study demonstrating the importance of APCs’ origin (donor or host) in initiating GVHD was done by Shlomchik et al. They showed that host APCs initiate CD8-dependent GVHD by directly presenting host Ag to donor T cells in a mouse model across only minor histocompatibility antigen s (miHAs) [97]. These host-derived APCs have been demonstrated to be dendritic cells (DCs), but not B cells [26]. Activation of host CD11c+DCs occurs rapidly after irradiation and could prime CD8 T cell s before their disappearance [121]. Later after transplantation, host APCs are replaced by donor APCs and donor APCs intensify GVHD once the disease is initiated by host APCs [69]. These donor APCs were demonstrated to be CD11c+ cells that efficiently cross-present host minor histocompatibility antigens to CD8 T cells [109].
On the other hand, how antigen is presented within MHC class II to donor CD4 T cells in CD4-dependent GVHD is not well known. It has previously been shown that MHC class II-bearing host hematopoietic APCs, particularly DCs [26], can initiate CD4 T cell-dependent GVHD [26, 104] and conventional DCs are the major donor APC subset presenting allogeneic peptides and maintaining GVHD [67]. Moreover, the study exploring molecular mechanisms demonstrated that the induction and maintenance of GVHD is critically dependent on RelB, the NF-kB/Rel family transcription factor, within both host and donor CD11c+DCs [66]. However, recent studies have shown that host hematopoietic professional APCs within lymphoid organs may have only a limited capacity to induce GVHD, and host DC s may not be required [60]. In contrast, nonhematopoietic host APCs within target organs are sufficient to induce GVHD [60]. Similarly, in the case of H–Y minor Ag incompatibility, either the donor hematopoietic-derived or the host nonhematopoietic-derived APCs is sufficient for inducing GVHD [105].
The Role of Innate Immunity
The significance of intestinal bacterial microflora in the pathogenesis of acute GVHD is well documented [7, 31, 86]. Current data implicate the innate immune response as being responsible for initiating or amplifying acute GVHD. Molecules such as bacterial lipopolysaccharide (LPS) that are released from the injured gut during the conditioning regimen activate innate immune receptors, including Toll-like receptor s (TLRs), and cause a cytokine storm, which favors the development of acute GVHD [44]. A variety of studies has suggested a role for intestinal bacterial microflora in the pathogenesis of acute GVHD, including the demonstration, in germ-free or completely decontaminated rodents, that the absence or complete growth suppression of intestinal bacteria prevents the development of acute GVHD [55, 106]. Hence, intestinal decontamination using broad-spectrum antibiotics before transplantation has been introduced, by some, as standard practice [4, 5].
A crucial role of the LPS-TLR4 pathway in the pathophysiology of GVHD has been demonstrated [21]. After allo-HCT, the translocation of LPS and microorganisms from the bowel lumen through the damaged intestinal mucosa to the circulation can occur. It has been shown that during GVHD, LPS stimulates the secretion of TNF-α by macrophages leading to increased GVHD mortality [82]. Using a mouse BMT model, Ferrara and colleagues demonstrated that the transplantation of donor BM cells, which are resistant to LPS stimulation, results in less severe GVHD [22] by attenuating early intestinal damage mediated by TNF-α. They also found that LPS antagonism, administration of a lipid-A analogue into a mouse BMT model, reduced serum TNF-α levels, decreased intestinal histopathology, and resulted in reduction of GVHD, but did not alter T-cell activity to host antigens [21]. Although the importance of the LPS-TLR4 pathway during GVHD is well defined as above, experiments with TLR4-deficient mice show that no difference in GVHD morbidity and mortality was observed in recipient mice lacking TLR4 as compared with wild-type mice, suggesting that TLR4 signaling is not absolutely required for GVHD [11]. Similarly, mutations in TLR4 have been shown to reduce GVHD risk [54], whereas another study reported an increased risk [27]. Thus, an association between TLR4 and GVHD has not been clearly defined.
Recent studies also suggested the critical roles of other TLRs in the pathogenesis of GVHD. In a murine GVHD model, reduced systemic GVHD leading to improved survival was observed in TLR9−/− allo-BM transplant recipients [11]. One of the most direct lines of evidence for the effects of TLRs on GVHD was derived by applying a TLR7 agonist to mouse skin before inducing GVHD. This resulted in massive T-cell infiltrates and GVHD pathology only at the site of pretreated skin [15]. In contrast, the TLR5 ligand, flagellin, and NOD2 have been shown to have an inhibitory effect on GVHD. Administration of highly purified flagellin into allo-BM recipient mice reduced GVHD by reducing early donor T-cell activation and proliferation [52]. Finally, increased GVHD was observed in NOD2-deficient allo-BMT recipient mice suggesting that NOD2 regulates the development of GVHD through suppressing the function of APCs [87]. In the clinical setting, polymorphisms of the genes encoding NOD2 are associated with a higher GVHD incidence [50].
It is important to mention that despite the prominent role of the innate immune system in the pathogenesis of GVHD, T cells can still be activated and GVHD can still occur in the absence of appropriate TLR signaling [64].
27.4.1.2 Phase II: Activation Phase
This phase represents the core of the GVH reaction, where donor T cells proliferate and differentiate in response to host APCs. Inflammatory cytokines and microbial products generated in Phase I augment this activation at least in part by increasing the expression of costimulatory molecules [29]. Donor T cells are critical in the induction of acute GVHD because depletion of T cells from the bone marrow graft effectively prevents GVHD [58] but at the expense of an increase in marrow graft rejection and leukemic relapse [37]. Recent clinical studies confirm experimental data demonstrating that the severity of GVHD correlates with the number of donor T cells transfused [57].
MHC class II differences between donors and recipients stimulate CD4 T cells, whereas MHC class I differences stimulate CD8 T cells [59, 99]. In the majority of HLA-identical HCTs, both CD4 and CD8 subsets respond to minor histocompatibility antigens and can cause GVHD. Thus both donor CD4 and CD8 T cells have crucial roles in the pathogenesis of GVHD.
Acute GVHD appears to be primarily a Th1 -driven process with massive release of IFN-γ, IL-2, and TNF-α and these cytokines have been implicated in the pathophysiology of acute GVHD [7, 29].
IL-2 production by donor T cells remains the principal target of many current clinical therapeutic and prophylactic approaches to GVHD. Monoclonal antibodies (mAb) against IL-2 or its receptor prevent GVHD when administered shortly after the infusion of T cells [30, 42]. Cyclosporine and FK506 dramatically reduce IL-2 production and effectively prevent GVHD [91]. But emerging data indicate an important role for IL-2 in the generation and maintenance of CD4+CD25+ regulatory T cell s (Tregs), suggesting that prolonged interference with IL-2 may have an unintended consequence of preventing the development of long-term tolerance after allogeneic HCT [119].
IL-15 is another critical cytokine in initiating allogeneic T-cell division in vivo, which has similar biological activities to IL-2, and has been identified as an indispensable costimulator in an experimental mouse model of skin GVHD [77, 78]. In humans, elevated serum levels of IL-15 are associated with acute GVHD [61].
IFN-γ has multiple functions and can either amplify [79] or reduce GVHD [9, 116] depending on the timing of its production. IFN-γ can have immunosuppressive effects at early time points after HCT but can exacerbate disease via its pro-inflammatory properties at later stages [7, 29]. IFN-γ may amplify GVHD by increasing the expression of molecules including adhesion molecules, MHC proteins, and chemokine receptors [29]. It also primes macrophages to produce pro-inflammatory cytokines and nitric oxide (NO) in response to LPS [82]. By contrast, IFN-γ may suppress GVHD by hastening the apoptosis of activated donor T cells [92].
Th2 -type cytokines can reduce acute GVHD [112] as mice receiving donor Th2-type cells are protected from GVHD [33]. Moreover, donor T cells that lack the four classical Th2 cytokines (IL-4, 5, 9, and 13) enhance T-cell proliferative responses and aggravate GVHD [102].
Th17 cells have been shown to have a direct role in GVHD pathogenesis. It has been shown that IL-17−/− donor T cells augmented Th1 differentiation and exacerbated acute GVHD [117] and adoptive transfer of in vitro differentiated Th17 cells induced lethal acute GVHD [13], although other studies have shown that an absence of IL-17 production by donor cells markedly impairs the development of CD4-mediated acute GVHD [56].
Genetic polymorphisms in TNF-α, IL-10, and IFN-γ have been linked to increased risk and severity of GVHD [14, 65, 71].
Subpopulations of regulatory cells can prevent GVHD. CD4+CD25+ Treg cells can suppress the proliferation of conventional T cells and prevent acute GVHD in animal models when added to the donor inoculum containing alloreactive T cells [20, 46, 103]. Donor NK cell s [2] and NKT cells of both the host and donor have been shown to suppress acute GVHD [120]. As well, CD3+CD4−CD8− double-negative regulatory T cell s, which specifically suppress CD8 and CD4 T cells that are primed against the same alloantigen, inhibit GVHD [76, 118].
27.4.1.3 Phase III: Effector Phase
The final effector phase of acute GVHD is characterized by cell damage via cellular mediators including cytotoxic T lymphocytes (CTLs) and NK cells and soluble inflammatory mediators including TNF-α, IFN-γ, IL-1, and NO [29, 31]. Three cytolytic pathways have been identified as important to GVHD: the perforin/granzyme B pathway, the Fas/FasL pathway, and direct cytokine-mediated injury [8, 44]. The soluble and cellular mediators synergize to amplify local tissue injury and further promote inflammation and target tissue destruction.
Cellular Effectors
The cellular effectors of acute GVHD are primarily CTLs and NK cells [29]. Donor cells from perforin -deficient mice can mediate GVHD but the onset of clinical manifestations is significantly delayed [3]. The result using granzyme B (gzm)-deficient mice showed a significant impairment in class I-dependent GVHD mediated by gzm B−/− CD8+CTL, whereas class II-dependent GVHD was not altered using gzm B−/− CD4+ effectors, suggesting that granzyme B plays a significant role in acute GVHD mediated by CD8+, but not CD4+ CTL [39]. Similarly, when mutant mice deficient in FasL are used as donors, GVHD occurs in an attenuated fashion [3]. Interestingly, the Fas pathway is important in the development of hepatic and cutaneous GVHD [3, 40]. When FasL-deficient mice are crossed with those having perforin knockout, the use of lymphocytes from these donors further diminishes but does not abrogate GVHD [8]. Currently, the Fas–FasL pathway is thought to be involved in skin and liver GVHD as well as systemic GVHD, whereas only some studies show evidence for involvement of the perforin–granzyme pathway in systemic GVHD and not target-organ GVHD [107].
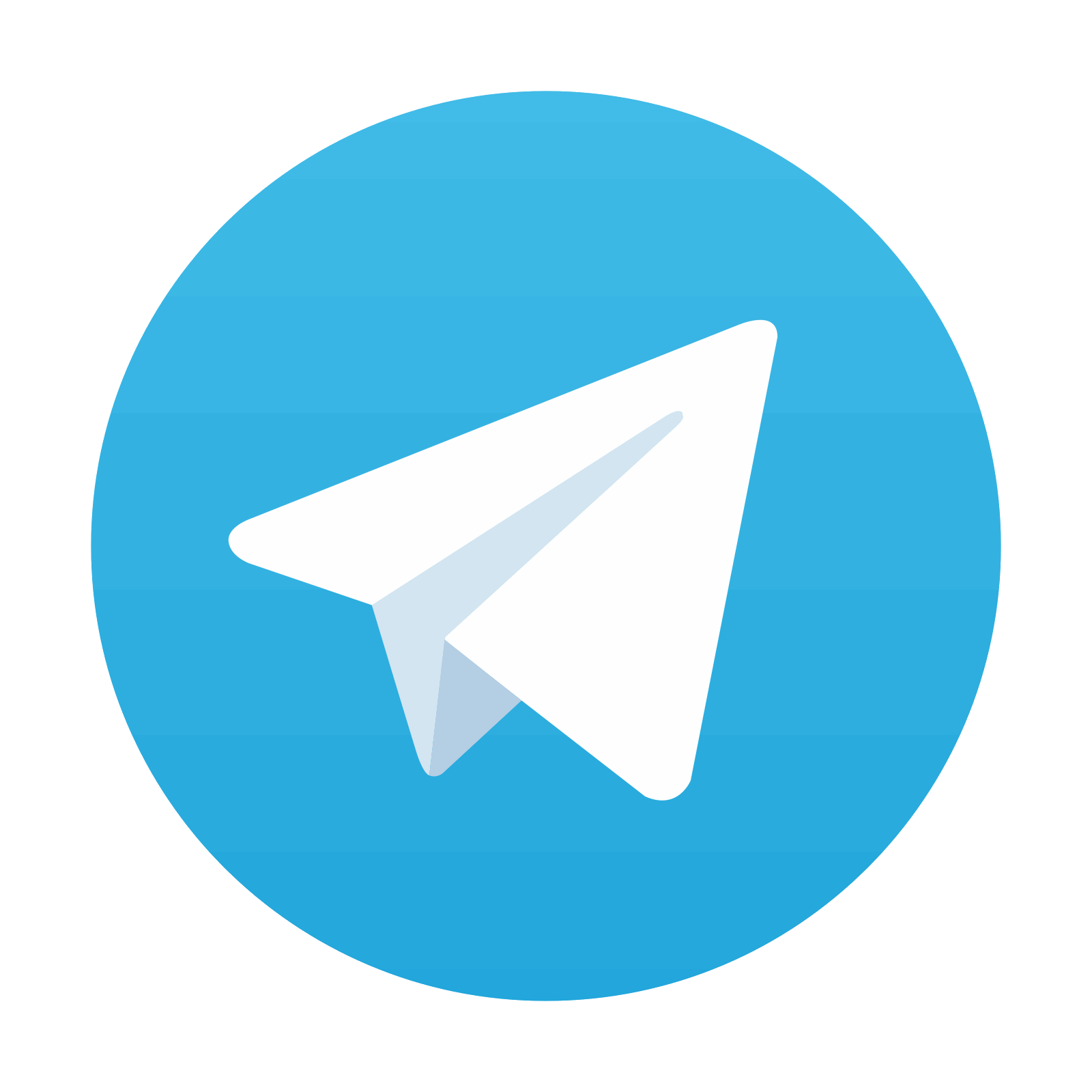
Stay updated, free articles. Join our Telegram channel

Full access? Get Clinical Tree
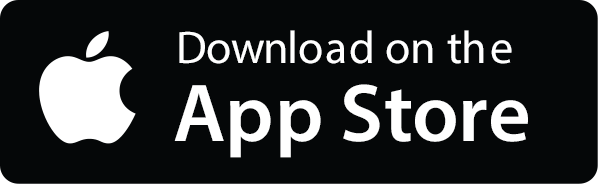
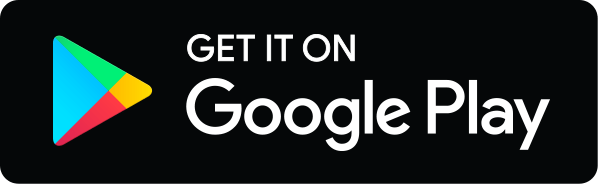
