Advantages
• Becoming more widely available
• Wide variety of tissue options
• Decreases the morbidity of the reconstruction by avoiding graft harvest
• Decreased operative time, no autograft harvest is required
• The allograft makes a single-staged procedure possible in large bony defects
Disadvantages
• Expensive
• Slower and incomplete incorporation
• Risk of disease transmission
• Risk of immunologic rejection and subsequent prolonged inflammation
• Biomechanical properties of the tissue may be compromised due to the sterilization techniques
• Inferior results found with objective stability testing compared to autografts
The increased availability of allografts in the United States has led to a significant increase in the utilization of these tissues in orthopaedic surgery. The American Academy of Orthopaedic Surgeons (AAOS) asserts that over five million musculoskeletal allografts have been utilized by surgeons in the past decade, while the data from the American Association of Tissue Banks (AATB), a voluntary organization that sets accreditation standards for tissue banking, reports that demand for musculoskeletal grafts has grown from nearly 700,000 grafts in 2001 to approximately 1.5 million distributed in 2007. Allografts are frequently used in revision ACL reconstruction, especially if autograft options are limited or compromised by the initial procedure. The Multicenter ACL revision study (MARS) demonstrated that 54 % of surgeons used an allograft at the time of revision reconstruction compared to only 27 % who utilized an allograft at the time of the initial reconstruction [3]. Within the MARS cohort, 50 % of the allografts were BTB, followed by tibialis anterior (23 %), Achilles tendon (12 %), and tibialis posterior (11 %) [3]. Other available options include the quadriceps tendon (QT), hamstring tendons, peroneus longus tendon, and fascia lata. The increased utilization of allograft for revision surgery can likely be attributed to a number of different factors, including more effective sterilization techniques, better organization and distribution of the tissues, and increased confidence in the strength and stability of the grafts [4–6]. Allografts are often appealing during revision ACL reconstruction because they avoid potential complications associated with hamstring or patellar tendon graft harvests, including donor site pain, patellar fracture, patellar tendon rupture, saphenous nerve injury, and persistent extensor mechanism or hamstring weakness [7–15]. In addition, large allografts are readily available and can fill expanded tunnels in the setting of revision ACL surgery, thereby allowing a single-stage reconstruction and avoiding the need for bone grafting and a staged procedure. Allografts can be harvested with a bone block as well (AT, BTB, or QT), which may allow for increased flexibility in revision cases where significant bone loss is encountered and allow for bone-to-bone fixation. Nevertheless, the benefits of allografts must be weighed against the potential disadvantages, including an increased cost, limited availability, slower graft incorporation, and the possibility of disease transmission or an immunologic response to the graft [16]. Most importantly, however, the increased rate of failure with allograft ACL reconstruction in a young and active population is a critical consideration, and may be of even greater consequence in a population that has already experienced a previous failure [17]. In this regard, it has been the senior authors’ preference to avoid the use of allograft for revision ACL reconstruction in this population, as the surgeon-controlled variables of technical accuracy and favorable biological properties of the graft should be prioritized.
Disease Transmission
One of the major concerns regarding the use of allografts is disease transmission. The true incidence of infection related to allograft knee surgery has yet to be determined. Nevertheless, the reported incidence is significantly less than 1 % (0.0004–0.014) [18, 19]. Despite a low incidence, however, there have been reports of transmission of human immunodeficiency virus (HIV), hepatitis B and C viruses (HBV and HCV), group A streptococcus, and Clostridium species [20–25]. The overall risk of viral transmission is lower and most cases have occurred prior to the discovery of the pathogen or before effective screening techniques were available to identify the pathogen. Currently, it is estimated that the risk of contracting viruses like HIV and HCV from an allograft is on the order of 1 in 1,667,600 [26].
Screening and Sterilization Techniques
All cells or tissues that are intended for transplantation into a human recipient are under strict regulations and any institution involved with recovering, processing, or storing these tissues must register with the FDA’s Center for Biologics Evaluation and Research and is strongly encouraged to seek accreditation from the Board of Governors of the AATB [18]. The FDA and the AATB mandate that all graft tissue be screened for HIV 1 and 2 antibodies, HIV-1 DNA by polymerase chain reaction (PCR), HBV surface and core antigen, HCV antibody, human T-cell lymphotropic virus types 1 and 2 antibodies, and syphilis antibodies. Additionally, the development of newer testing methods such as advanced nucleic acid testing (NAT) have increased safety by allowing for screening for human transmissible spongiform encephalopathies and has significantly decreased the window period associated with the conventional antigen and antibody testing [19, 27–29].
Prior to procurement of the tissue, allograft donors are initially screened with an exhaustive review of the donor’s medical and social history as well as with postmortem examinations for signs of infection. Typically, donor tissue is procured under aseptic technique in the operating room. Blood as well as samples from the allograft tissue are cultured as part of the initial screening process. Several sterilization techniques have been used in an attempt to eradicate bacteria, viruses, and spores from the donor tissue. There is no single standardized approach to sterilizing the graft tissue. Currently, there are two commonly employed processes for sterilization: gamma irradiation (GI) and chemical processing. GI can effectively be used to eradicate bacteria with doses of only 1.5–2.5 mrad. Unfortunately, it requires as much as 4.0 mrad to render HIV inactive and often even higher doses are necessary to eliminate spores [30]. Because GI results in the generation of free radicals that ultimately kill the pathogens, these free radicals can also affect the structural integrity of the graft itself. Fideler et al. studied the effects of GI on BTB allografts. The study found a 15 %, 24 %, and 46 % reduction in all biomechanical properties after exposure to 2.0, 3.0, and 4.0 mrad, respectively [31]. Ethylene oxide (EO) was shown to be an effective external sterilization technique, but had poor tissue penetration and had been associated with intra-articular reactions with chronic synovitis. Consequently, it has not been used in the past 10 years [18, 19, 29, 32]. There are several novel chemical sterilization processes available to process allografts. Some of the commonly used proprietary tissue sterilization techniques include Clearant Process (Clearant, Inc., Los Angeles, CA), Allowash XG (LifeNet, Virginia Beach, VA), Biocleanse (Regeneration Technologies, Inc., Alchua, FL), and Tutoplast (Tutogen Medical, Inc., Alchua, FL). While each manufacturer uses a slightly different technique, proprietary sterilization generally includes soaking the graft in a solution. The tissues are then placed in a centrifuge and spun with or without an agent such as H2O2 or alcohol. This may be followed by a second rinse and then the tissues are irradiated prior to deep freezing. Deep freezing has not been demonstrated to alter the biomechanical properties of the tissue, but it doesn’t substantially contribute to the sterilization process since some viruses, like HIV and HCV, are not destroyed at these temperatures. Unfortunately, no independent testing or validation of these proprietary sterilization techniques has been performed to characterize their efficacy or effect on the biomechanical integrity of allografts.
Healing and Incorporation of Allografts
Graft healing and biologic incorporation warrant significant consideration when comparing allografts to autografts for revision ACL reconstructions. Both graft types undergo the same healing process of “creeping substitution” that begins with an initial period of avascular necrosis followed by revascularization and host synovial cell proliferation. Jackson et al. found that the donor DNA was replaced by host DNA by 4 weeks as a result of this process [32, 33]. While the mechanisms of integration may be similar, the literature has demonstrated that allografts undergo this process at a much slower rate than autografts. This was again confirmed by the work performed by Malinin et al. [34]. This study evaluated nine ACL allografts and one autograft that were retrieved at autopsy or at the time of revision surgery to evaluate extent of graft cellular replacement and remodeling at different graft ages. The age of the specimens ranged from as early as 20 days to 10 years post implantation. The examination of the entire allograft 2 years after implantation revealed that the center portion of the grafts remained acellular. The study found only one sample to have complete cellular replacement at 3.5 years postoperatively. This led the authors to conclude that complete remodeling and cellular replacement will occur in allografts, but it may require 3 or more years for complete incorporation [34]. This delayed integration may contribute to the overall decrease in the biomechanical properties of the allograft. Jackson et al. [35, 36] demonstrated that autografts had better stabilization of AP translation, twice the maximum force to failure strength, an increase in cross-sectional area, and a more rapid conversion of large diameter collagen fibers to small diameter fibers. Ultimately, the allografts demonstrated a greater decrease in biomechanical properties, an overall slower rate of incorporation, and a prolonged inflammatory response when compared to the autografts [35, 36]. For these reasons, the senior authors have avoided the use of allografts for ACL reconstruction, particularly in the revision setting in which a recurrent failure may be catastrophic to an athletic career. In a population with a previous reconstructive failure, the technical graft position, graft fixation, time-zero biomechanics, and biology must be optimized to offer the greatest chances for a favorable structural and clinical outcome in a potentially adverse host environment.
Clinical Outcomes
Clinical studies evaluating revision ACL allograft reconstructions have demonstrated reasonable outcomes, though they remain inferior to autografts for primary and revision ACL reconstruction in a young, active population [17]. Several studies also support the basic science findings that allograft incorporation is delayed and the biomechanical properties of the allograft are inferior to those of autografts [34–36]. In a prospective study of revision ACL reconstruction, Noyes and Barber-Westin evaluated 65 patients who received BTB allografts and 20 patients with autogenous BTB grafts [37]. Overall, 33 % of the allografts failed compared to 27 % of the autografts. Additionally, KT-2000 testing showed that 53 % of the allograft cohort and 67 % of the autograft group had less than 3 mm of displacement [37]. Similarly, Grossman et al. [38] compared 30 revision ACL reconstructions that used allografts (29 BTB and 1 AT) with 6 patients who had BTB autografts transplanted at the time of the revision reconstruction. The allograft group demonstrated increased laxity during instrumented knee testing compared to autografts (3.21 vs. 1.33 mm, respectively) [38]. Uribe et al. [39] reviewed 54 patients who underwent revision ACL reconstruction. Of the 54 patients, BTB allografts were used in 35 % of the cases while autologous hamstring grafts were used in 65 %. There was no subjective difference found between the two graft types, but the allografts did demonstrate decreased stability on KT-1000 testing compared to the autografts [39]. Battaglia et al. [40] reported on 63 patients who underwent ACL revision reconstruction, 40 of which were performed with autologous grafts and 23 with allografts. The overall failure rate was 25 % in the autograft subset compared to 30 % for the allograft group [40]. Based on the currently available literature, allografts present a greater risk of structural failure after ACL reconstruction and the senior authors would not advocate their use in the revision setting unless other viable autograft options have been compromised.
Autografts (Table 8.2)
Table 8.2
Advantages and disadvantages of autografts
Advantages |
• Eliminates the risk of disease transmission |
• Eliminates the risk of graft immunologic rejection |
• Faster and more complete biologic incorporation |
• Decreases cost compared to allografts |
• Better results with objective stability testing when compared to allografts |
Disadvantages |
• Fewer tissue options available |
• Autograft tissue may not be available for revision reconstructions |
• Increased morbidity related to autograft harvest |
• Patient may refuse autograft harvest |
Despite the increasing utilization of allografts, autografts continue to be the graft of choice for primary and revision ACL reconstructions. Despite the donor site morbidity, autografts have considerable advantages over allografts. Autografts carry no risk of disease transmission or immunologic rejection. Additionally, they have a faster and more reliable biologic integration than allografts, which may be of particular importance in a revision surgery with an impaired healing environment in a previously repaired knee.
Currently, there are several options for autograft harvest sites for primary and revision ACL reconstructions. The most commonly used grafts are the BTB and hamstring tendons for primary ACL reconstruction, while the quadriceps tendon (QT) may be another favorable option for revision surgery in the setting of tunnel expansion or previously harvested grafts. Autologous grafts can be harvested from either the ipsilateral or contralateral limb depending on patient preference and what tissue was used during the primary reconstruction.
BTB Grafts
The central third of the patellar tendon along with bone plugs from the tibia and patella are considered the gold standard by many surgeons. Because of the presence of the bone plugs, BTB grafts allow for bone-to-bone healing within the tunnels and have been shown to provide a faster and more reliable incorporation of the graft than tendon-to-bone grafts [41–43]. Integration of the bone plug begins with osteonecrosis at the graft-tunnel interface. This is followed by creeping substitution of the host bone into the graft and rapid incorporation into the surrounding host bone. At 3 weeks, the bone plug is surrounded by dense fibrous tissue and by 6 weeks the bone plug has undergone complete integration into the host bone [42].
Another advantage of the BTB grafts is that they allow the flexibility to adjust the graft size to match the tunnel size. Depending on the size of the patellar tendon, up to a 12-mm width graft can be taken while still retaining an appropriate amount of tendon on either side of the harvest site. While size may be a limiting factor for the patellar harvest site, the tibial bone plug can be widened or lengthened to fill large bony defects that are often present in revision cases.
BTB autografts have some negative characteristics that have been cited in the literature, such as risk of graft-tunnel mismatch, anterior knee pain at the harvest site, and patellar fracture. Despite the ability to adjust the bone plug size to fit the defect, the BTB graft soft tissue component cannot easily be lengthened or shortened, unlike soft tissue grafts like hamstring and QT grafts. This mismatch may be particularly evident with previously prepared tunnels or when the femoral tunnel is placed in an anatomic position on the lateral wall of the femoral notch, which effectively reduces the intra-articular graft length compared to a more conventional over-the-top position. Therefore, if the graft is either significantly shortened or lengthened in comparison to the size needed for the reconstruction, the surgeon must be prepared with alternate fixation options to solve this graft-tunnel length mismatch [44–46].
Significant anterior knee pain is a well-known problem associated with BTB autografts, with occurrence rates as high as 40–60 % [47–52]. Although there are some conflicting results in the literature when comparing hamstring to BTB grafts regarding the incidence of anterior knee pain, the incidence of anterior knee pain is likely higher in the BTB group. Roe et al. [51], in a study with long-term follow up, noted that significant anterior knee pain persisted even at 7 years postoperatively and was more common and more severe in the BTB group compared to the hamstring autograft group [51]. Additionally, the incidence of donor site symptoms of any kind was more than doubled in the BTB group compared to the hamstring tendon group. The BTB group was also more prone to develop a slight extension deficit over time [51].
The risk of a patellar fracture is a rare complication that may occur in approximately 1 % of ACL reconstructions with a BTB autograft [53, 54]. The surgeon can decrease the risk of a patellar fracture by ensuring that the harvested bone plug is no more than half the length of the patella and by avoiding cross-hatching and making angled cuts with depths no greater than 10 mm. Most commonly, the fractures occur in the vertical orientation and ultimately do not disrupt the extensor mechanism. These fractures can usually be treated conservatively with nonoperative management. Occasionally, transverse fractures occur, but are usually the result of postoperative trauma. These fractures generally require repair because the fracture is at risk of displacement and compromising the function of the extensor mechanism.
Special consideration must be given in pediatric patients before utilizing a BTB autograft for a reconstruction. There is concern that using a BTB graft in a patient with open physes may cause an angular deformity due to placement of the bone plug across the physis or recurvatum deformity secondary to tubercle apophyseal injury. Consequently, the location of the graft within the physis is of significant importance because a centrally positioned physeal defect may result in premature closure but is much less likely to cause an angular deformity. There is less of a risk of this deformity in the tibia where the graft tunnel is placed more centrally in the physis, whereas, the femoral tunnel is often drilled more obliquely. In addition, tunnel obliquity affects more total volume of the physis, which can result in a larger disruption of the growth plate. A BTB graft is at a greater risk of a large physis disruption because of the thickness of the graft ends. An animal study demonstrated that the larger the cross-sectional area of the physis that is affected, the greater the chance of a growth disturbance. The same study found that there is an increased risk of partial physeal closure when greater than 7 % of the physis is disrupted.
Re-harvested BTB has also been used in revision ACL reconstructions. Currently, there are conflicting data regarding the success of this graft in revision ACL reconstruction. Colosimo et al. [55] found that 11 patients had good or excellent results and 2 patients had fair results after undergoing a revision ACL reconstruction with a re-harvested BTB graft. Mean follow up was 29.4 months and postoperative KT-1000 testing demonstrated an average side-to-side difference of 1.92 mm. There was no loss of range of motion in any of the patients and only 1 patient reported moderate patellofemoral problems. Based on these results, the authors concluded that a reharvested BTB graft is a viable option for revision ACL reconstruction [55]. O’Shea and Shelbourne also reported good subjective and objective results in a group of 11 patients with a mean follow up time of 49 months [56]. In contrast, Kartus et al. [57] demonstrated a higher rate of complications and poorer functional scores in the patients who underwent reconstruction with a re-harvested BTB graft in comparison to cases where the contralateral patellar tendon was used for graft harvest. Of the 12 patients with a re-harvested BTB graft, 1 had a patellar fracture 2 weeks postoperatively and another suffered a patellar tendon rupture 6 months postoperatively [57]. Liden et al. [58] noted similar results in a 10-year follow up study of 14 patients with re-harvested BTB reconstruction. MRI studies at 10 years following the re-harvesting procedure demonstrated that the patellar tendon at the donor site had not normalized. Additionally, Lysholm, IKDC, and KT-1000 scores as well as single leg hop and knee-walking testing noted no significant difference between results at 2 years and 10 years postoperatively. Overall, the results were considered to be poor at both postoperative timepoints [58]. As with the study by Kartus et al. [57], the two major complications noted in this cohort were one patellar fracture and one patellar tendon rupture.
The senior authors have favored the use of native BTB graft when available for revision ACL reconstruction. This graft is particularly favorable in the setting of well-positioned tunnels without considerable expansion or in the setting of newly prepared tunnels in a primary or staged reconstruction.
Hamstring Grafts
Hamstring autograft has been used successfully in revision ACL reconstructions for many years. In a recent study, Salmon et al. [59] reported on 57 revision ACL reconstructions using 4-strand hamstring autografts with an average of 89 months of follow up. Of the 50 knees reviewed, 5 (10 %) had objective failure of the graft. In the remaining 45 patients knee function was normal or nearly normal in 33 cases (73 %). Fifty percent of the knees had less than 3-mm of translation with the remaining 50 % having 3–5 mm [59].
Typically, both the gracilis and the semitendinosis tendons are harvested and then doubled and combined to create a 4-strand graft, though some authors have noted using 5- and 6-strand grafts to improve the graft strength when sufficient length is available. Currently, there are numerous fixation devices available for soft tissue grafts such as cortical suspensory fixation devices and aperture interference fixation devices.
One significant advantage to using the hamstring graft is the avoidance of the potential side effects inherent with BTB grafts, especially anterior knee pain and patellar fracture. This may be particularly important in patients who perform a significant amount of kneeling or squatting during work. In a 9-year follow up study, Wipfler et al. [60] found that hamstring autografts had better kneeling, knee walking, single leg hopping, and IKDC scores compared to BTB grafts. Leys et al. [61] found that the hamstring autograft group had better results regarding the incidence of osteoarthritis, motion loss, single leg hop, and kneeling pain postoperatively, but the study found no difference in IKDC scores [61].
The most commonly reported disadvantages to hamstring grafts are related to size, the propensity of the graft to develop laxity, the ACL agonist function of the hamstring muscle-tendon units, and the delayed healing of tendon-to-bone compared to bone-to-bone. Hamstring grafts can often be small and cannot be adjusted or customized to match existing tunnel dimensions, which are frequently greater than 10 mm in diameter. In the revision setting, incomplete tunnel fill with these grafts is unfavorable and likely increases the risk of failure and incomplete healing and the tendon-bone interface. The occurrence of laxity is generally related to the number of graft strands used in the reconstruction. In 2010, a systematic review found, in two studies, that 2-strand hamstring grafts developed laxity over time when compared to BTB grafts [62]. Whereas, only 1 of the 4 4-strand hamstring grafts analyzed was found to have a significant difference in laxity when compared to BTB grafts.
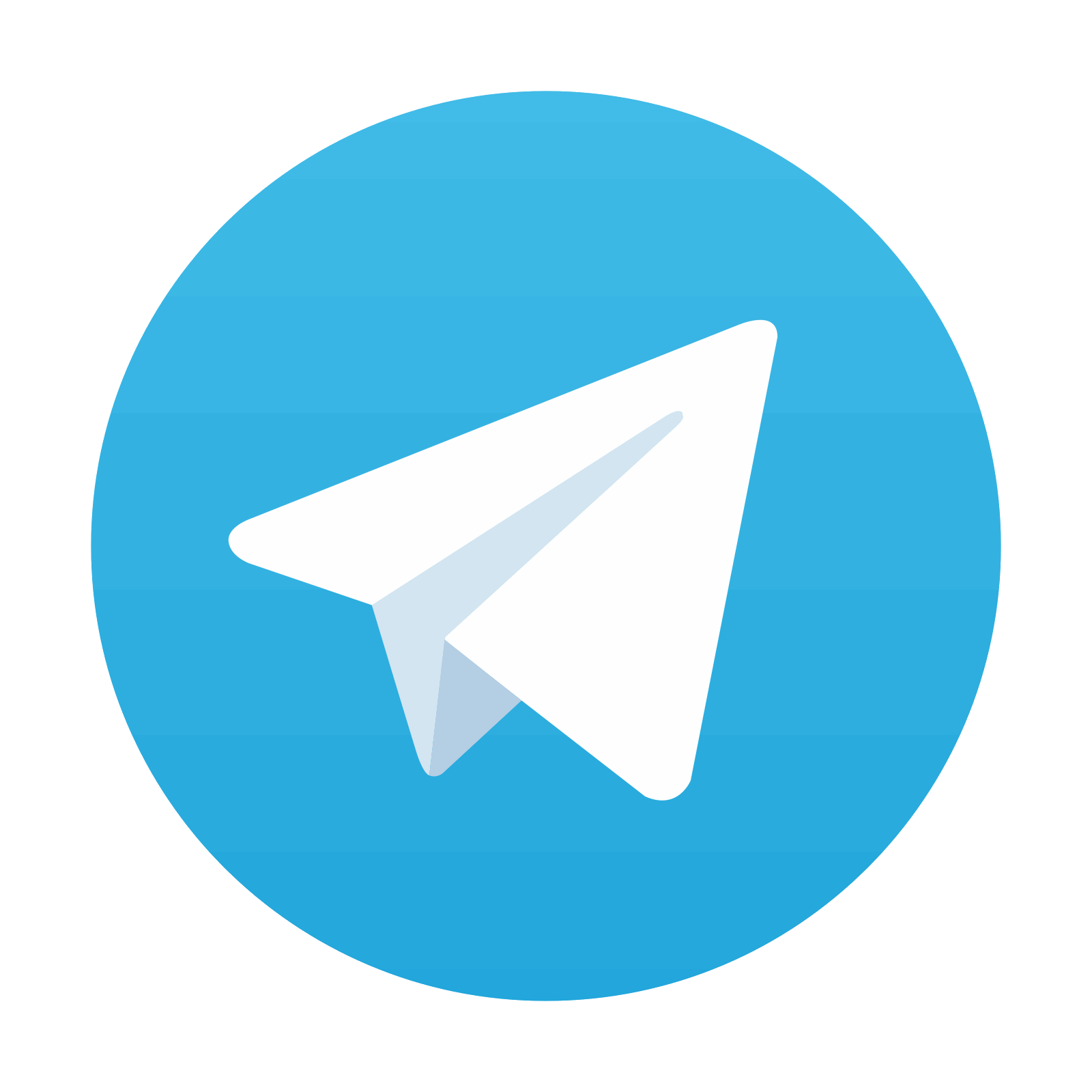
Stay updated, free articles. Join our Telegram channel

Full access? Get Clinical Tree
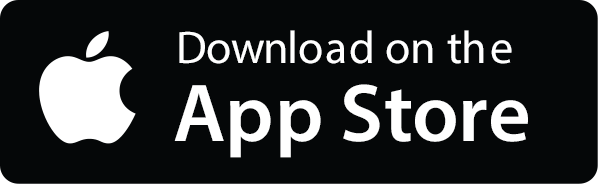
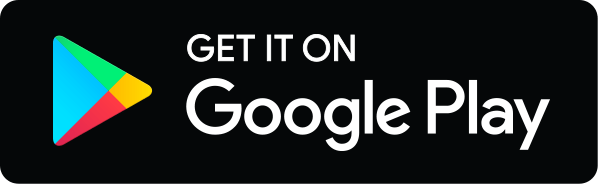