Lasers for the treatment of vascular lesions are continually improving using the principles of selective photothermolysis.
Important variables to consider in the treatment of vascular lesions are size, depth, and composition.
Effective treatment of vascular lesions requires tailoring the light wavelength, fluence, and pulse duration to the specifics of the targeted lesion.
Introduction
Lasers can be used to target and destroy vascular lesions. Diverse arrays of lasers effective in treatment are currently available. |
A large number of lasers and intense non-coherent pulsed light devices are available for the treatment of vascular lesions. They provide for the resolution or improvement of vascular lesions that were previously untreatable. New devices have been developed and existing devices are continually being improved to increase their ability to target vessels while decreasing the risk of adverse reactions. We review here vascular lesions and the lasers commonly utilized for their treatment.
Principles of Laser Treatment for Vascular Lasers
Selective photothermolysis describes the selective interaction of light energy with selected targets within the skin. Chromophores are molecules or structures that preferentially absorb specific wavelengths of light. Thermal relaxation time is the time it takes for a target structure to dissipate 50% of the absorbed energy into surrounding tissue and is an important consideration during the selection of treatment parameters. In the visible and near-visible spectrum, increasing depth of penetration into tissue correlates with increasing wavelengths Pulse duration affects selectivity of treatment as well as depth and risk of adverse results. Epidermal cooling helps to minimize collateral thermal damage, but has to be tailored to the treatment parameters and lesion location to prevent cryogenic damage or pain. |
Selective Photothermolysis
In 1983, Richard Rox Anderson and John Parrish proposed the theory of Selective Photothermolysis.1 The selective destruction of vascular lesions with light energy is based on this principle. This effect requires a wavelength of light to penetrate to at least the depth of the target and be absorbed significantly more by that target than the surrounding chromophores that are also in the light path. In addition, the target must absorb enough energy to irreversibly damage it, but cool quickly enough so that the heated target does not cause thermal damage to the surrounding tissue. The target (chromophore) for vascular lasers is hemoglobin. The wavelength of light that it preferentially absorbed depends on whether it is bound to oxygen or not and whether the ferrous iron in the heme group is oxidized to ferric iron or not.
Lasers available today for the treatment of vascular lesions span a large spectrum of individual wavelengths from 532 to 1,064 nm with non-coherent pulsed light sources spanning a larger spectrum. Choosing the appropriate wavelength involves selecting a wavelength with deep enough penetration that is primarily absorbed by the vascular target. Within this range, shorter wavelengths scatter more quickly and penetrate less deeply than longer wavelengths which can reach several millimeters into the dermis before loss to scattering renders it useless. Oxygenated hemoglobin (oxyhemoglobin) is normally the most abundant form of hemoglobin and has three peaks of absorption (418, 542 and 577 nm). Deoxygenated hemoglobin (deoxyhemoglobin) has an absorption peak of 755 nm that is well targeted by the 755 nm wavelength alexandrite laser. The major chromophores competing for absorption in the skin are melanin, water, and fat.
Melanin is primarily present in the epidermis and may absorb incident laser light. This may reduce the effective energy delivered to the vascular target and possibly cause direct thermal damage to the epidermis. Occurring between 532 and 1,064 nm, absorption by melanin decreases with longer wavelengths from ultraviolet (<400 nm) through the visible (400–760 nm) spectrum (Fig. 1). Longer visible and near infra-red wavelengths are absorbed less by epidermal melanin and penetrate deeper into the dermis and blood than shorter visible and ultraviolet wavelengths.2
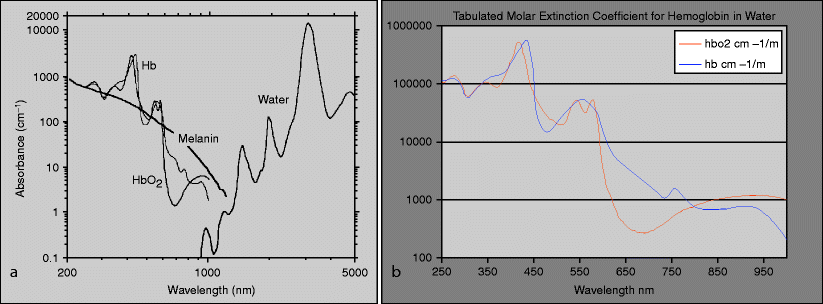
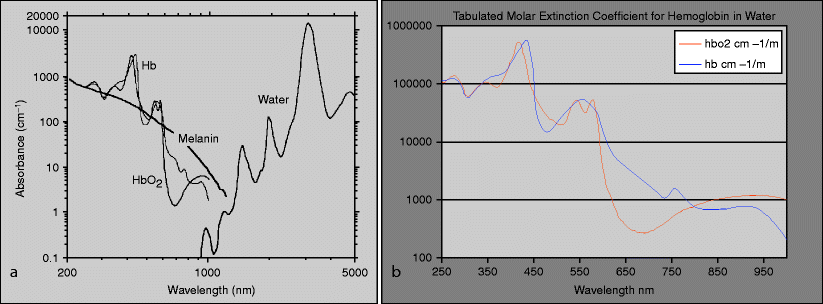
Fig. 1
Absorption curve for (a) hemoglobin, melanin and water, and (b) for oxygenated and deoxygenated hemoglobin
Pulse Duration
As developed in the theory of selective photothermolysis, Anderson and Parrish describe the selective targeting of a chromophore based on the target size which determines the optimal length of the laser pulse. The thermal relaxation time was defined as the time it takes for a target structure to dissipate 50% of the absorbed energy into surrounding tissue. This can be approximated by the square of the diameter of the target structure. High enough fluences with pulse durations short enough to limit diffusion of heat from the target vessels will cause photocoagulation.3 Excessively long pulse durations, longer than the thermal relaxation time, can lead to diffusion of thermal damage to surrounding tissue. In contrast, excessively short durations can cause focal boiling of the blood which may lead to cavitation-induced vessel disruption. This can produce excessive purpura without necessarily causing elimination of the vessel.
Epidermal Temperature Control
The epidermis is particularly sensitive to thermal damage. Heat transfer to the epidermis during laser therapy can occur by several mechanisms: direct absorption of light energy, back scatter of light from deeper tissue, and conduction of heat produced in the dermis. Selective cooling of the epidermis before, during, and/or after treatment allows for production of higher temperatures in the target structures without damage to the epidermis. Current methods include: direct contact (conduction), forced cold air (convection), and cryogen spray (conduction and evaporation).
Lasers for Treatment of Vascular Lasers
The frequency-doubled Nd:YAG, KTP filtered laser produces laser light of 532 nm and can be used to target very superficial vessels. Pulsed dye lasers produce light that penetrates deeper than frequency-doubled Nd:YAG and is more selectively absorbed by hemoglobin. These lasers have become a mainstay of vascular lesion treatment. Alexandrite lasers produce 755 nm wavelengths light that can better target deoxygenated hemoglobin. Neodymium:Yttrium-Aluminum-Garnet lasers can be used to treat vascular lesions, but are less selective and have a higher risk of causing dermal damage. A combination of PDL and Nd:YAG laser has been developed that may more selectively target vessels that have both superficial and deep components. Intense pulsed light devices produce high-energy broad spectrum non-coherent light that can be selectively filtered to target vascular lesions. |
Frequency-Doubled Nd:YAG (Potassium-Titanyl-Phosphate [KTP])
Frequency-doubled neodymium:yttrium-aluminum-garnet lasers (Nd:YAG) use potassium-titanyl-phosphate (KTP) crystals to double the frequency, which halves the wavelength from 1,064 to 532 nm. Oxygenated hemoglobin absorbs the light of this wavelength about as well as it absorbs the light of 585 nm wavelength (Fig. 1). The shorter wavelength is effective for destruction of the most superficial vessels though melanin also absorbs this wavelength well. Efficient epidermal cooling is necessary when using these devices, especially in darker skin types where epidermal melanin is more of an issue.
Pulsed Dye Lasers (PDL)
The flash lamp-pumped pulsed dye laser (PDL) was the first laser developed for selective photothermolysis of vascular lesions.1,4 It was initially designed to produce 577 nm light to correspond with one of the oxyhemoglobin peaks5 (Fig. 1). The wavelength was increased to 585 nm to increase depth of penetration, improving efficacy for the treatment of deeper vessels. The original pulsed dye lasers had short pulse widths of 0.45 ms, whereas the next generation of PDLs had the ability to deliver high frequency pulses that could be combined into longer combined pulse widths of 1.5 ms. The longer pulse widths allow treatment of larger vessels with less extravasation of blood and therefore less purpura. Although longer wavelengths allow deeper penetration, Hb absorption begins to drop off rapidly. The next generation of PDLs is also able to produce light with wavelengths of 585, 590, 595, or 600 nm. The most recent versions of pulsed dye lasers have only the 595 nm wavelength but have adjustable pulse widths ranging from 0.45 to 40 ms to provide optimal treatment parameters for various vessel sizes.
Alexandrite Laser
Bluish vascular lesions often extend deeper and contain more deoxyhemoglobin. PDLs are not very effective against these lesions, because their wavelengths often do not penetrate deeply enough and are not absorbed by deoxygenated hemoglobin as well as they are by oxygenated hemoglobin. One of the most recent advancements in the development of vascular lasers is the utilization of a deoxygenated hemoglobin absorbance peak at 755 nm by the long pulsed alexandrite laser.6 Initially, the long pulsed alexandrite laser was used for hair removal, but was found to be effective against violaceous deeper vascular lesions.7 This is because, in addition to being well absorbed by deoxygenated hemoglobin, long pulsed alexandrite lasers produce a longer wavelength over longer pulse widths that allow the light to penetrate further and to target larger vessels.
Neodymium:Yttrium–Aluminum–Garnet (Nd:YAG)
Neodymium:yttrium-aluminum-garnet (Nd:YAG) lasers produce light at 1,064 nm which penetrates deeper than shorter wavelengths. However, at this wavelength, oxyhemoglobin no longer absorbs the energy better than the surrounding dermis. These lasers can be effective, but risk of scarring is significant at fluences high enough to effect vascular damage so these lasers are less often used.8 Fluences and pulse width used at this wavelength are dependent on vessel size, location, and the oxygenation of hemoglobin. Vessels with larger diameters require longer pulse widths. Conversely, small vessels need shorter pulse widths, but require higher fluences, as there is less chromophore (Hb) present.
Combination PDL and Nd:YAG Laser
Methemoglobin is hemoglobin in which the ferrous iron of the heme group has oxidized to ferric iron. Unlike oxyhemoglobin and melanin, methemoglobin has increased absorption with increasing wavelength from 700 to 1,000 nm and is well targeted by Nd:YAG laser light[reference/spectrum]. It has been shown that pulsed dye lasers are able to induce the transformation of oxyhemoglobin to methemoglobin resulting in a more selective target for an Nd:YAG laser.9 Recently, a device combining a PDL (595 nm) and Nd:YAG (1,064 nm) was developed utilizing this concept.
In addition, the thrombus that can be formed by PDL energy also absorbs 1,064 nm light better.9,10 Because of the increased absorption of 1,064 nm by the methemoglobin and thrombus, lower fluences of the Nd:YAG produce vessel specific damage with decreased thermal damage to surrounding tissue.
PDL light only penetrates approximately 0.75 mm into the skin so. For lesions with deeper components, it is necessary to allow the PDL formed products time to flow deeper into the lesion before illumination with the deeper penetrating Nd:YAG laser. The combination therapy works best for superficial discrete telangiectasias with vessels in the 0.2–1.2 mm diameter range. Though PDL remains the treatment of choice for vascular birthmarks, the PDL/Nd:YAG combination may be helpful for lesions resistant to PDL alone. As we get more proficient with the use of the dual wavelength modality, we can use it more efficaciously.
Intense Pulsed Light (IPL)
Intense Pulsed Light (IPL) devices produce high-energy, non-coherent light. Their output covers a broad range of the light spectrum, often ranging from near 500 nm to around 1,200 nm with pulse widths of several milliseconds. Cut-off filters are used to limit the spectrum of the incident light to the desired wavelengths. For treatment of vascular lesions, wavelengths shorter than 580–590 nm are generally filtered out.11 Newer devices filter out the longer infra-red wavelengths, reducing many of the adverse effects of earlier devices.11 These devices usually have larger application tips which cover larger surface areas and are well suited to broader treatment areas.
Output varies by wavelength and by device so parameters tend to be device-specific. IPL devices can be effective against capillary malformations, telangiectasias, and small cherry angiomas.12 Adverse effects include post-inflammatory hyper- or hypopigmentation, blistering, and scarring.
Vascular Lesions and Laser Treatment Options
Congenital capillary malformations (e.g., port wine stains) are the most common type of vascular malformation and can often be effectively treated with lasers. Hemangiomas are vascular neoplasms that often partially resolve, but can cause functional and developmental problems in addition to leaving lasting scars. Telangiectasias represent small superficial dilated capillaries and post-capillary venules with thickened walls. Venous lakes are late developing venous ectasias that can vary greatly in size and depth. Laser treatment is often effective, but needs to be tailored to the depth and size of the lesion. |
Capillary Malformations (e.g., Port Wine Stains or Nevus Flammeus)
Capillary malformations are the most common type of vascular malformation. Also called a port wine stain (PWS) or nevus flammeus, they represent congenital developmental malformations of the superficial dermal capillaries. They are present at birth, grow as the child grows, and do not involute.
Treatment of port wine stains (PWSs) was initially performed with the continuous wave 488–514 nm argon laser.13,14 The excess heat deposition from this laser often leads to dyspigmentation and scarring. One of the main problems with the argon laser was that the pulse width could not be controlled. This often led to scarring from excess heat deposition. A laser with a longer wavelength and a controllable pulse width was needed. In an effort to overcome the inadequacies of the argon laser for treating these lesions, Richard Rox Anderson and John Parrish developed the theory of selective photothermolysis. This led to the PDL becoming the treatment of choice for these lesions. The PDL in its current reincarnation remains the gold standard.18 This laser selectively targets hemoglobin, and the pulse width is more closely matched to the thermal relaxation time of the targeted vessels in PWSs.15,16
Most PWSs start as pink macules with tiny vessels, but with time these lesions, usually found as hypertrophy and in the vessels, become larger and tortuous.17 In addition, more of the hemoglobin in larger, older lesions tends to be deoxygenated and are not targeted as well by PDLs. For these reasons PWSs are generally best treated with PDLs earlier in the pink macular stage, as often seen in infants. More mature hypertrophic bluish PWSs often require longer pulse-widths and even longer wavelengths. Successful treatment generally requires repeated treatments especially for larger lesions and those on the distal extremities.18 As discussed above, longer wavelength PDLs can treat deeper vessels in the lesion and may show improved rates of clearance, though the decreased absorption by hemoglobin necessitates higher fluences which may be more likely to produce post-inflammatory pigment changes.
Most patients see significant improvement, but total removal of the lesion is often not possible. Lesions in newborns tend to respond faster and more completely than those in older patients. There is much less melanin in neonatal skin competing as a chromophore for the absorption of the laser and the lesions tend to be thinner and much smaller than they will often become. All these features make PDLs more effective against earlier lesions than they are against later lesions.
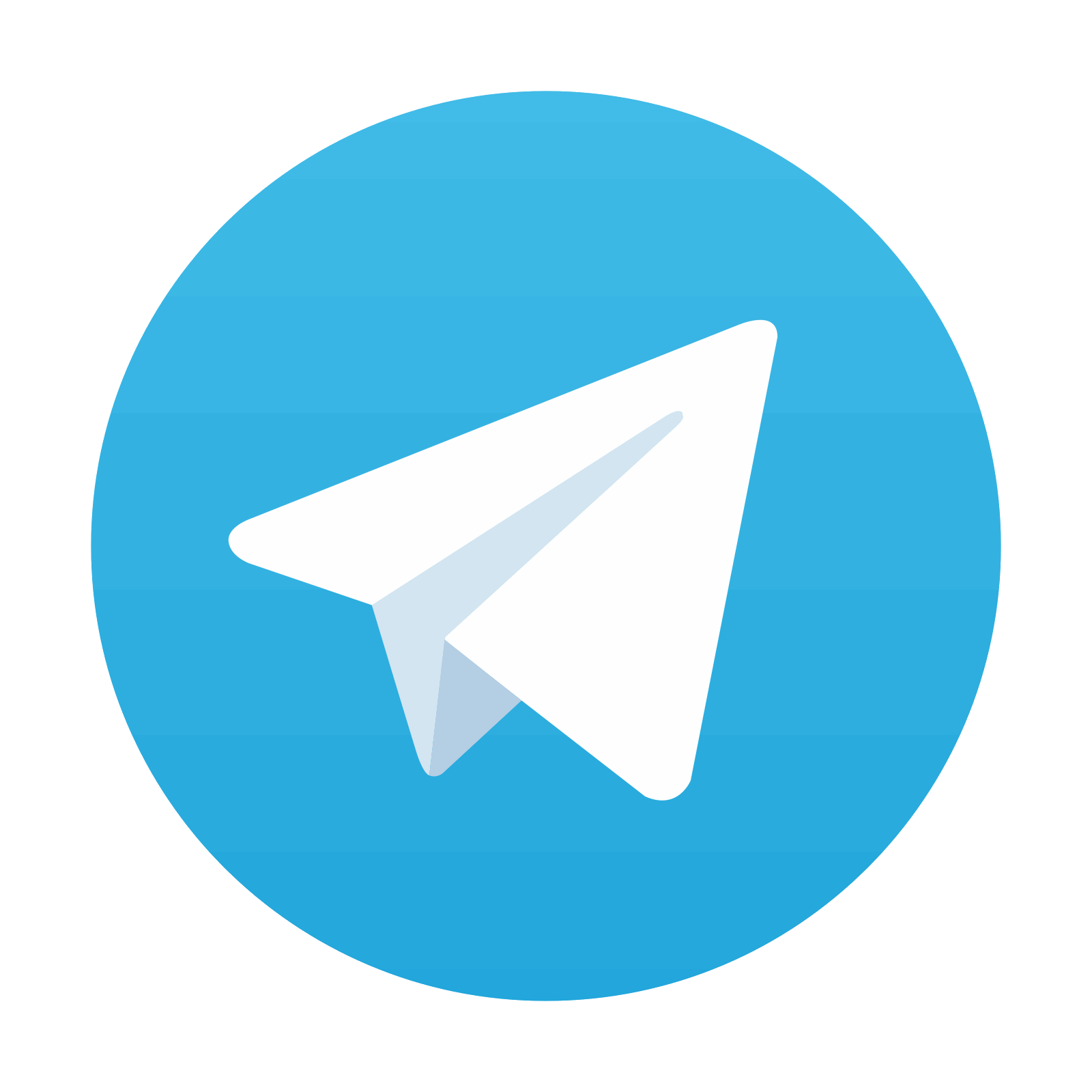
Stay updated, free articles. Join our Telegram channel

Full access? Get Clinical Tree
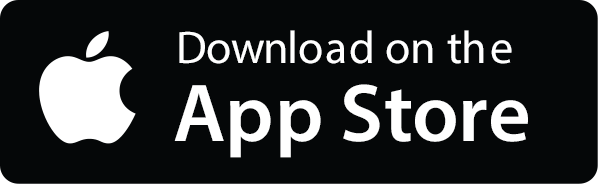
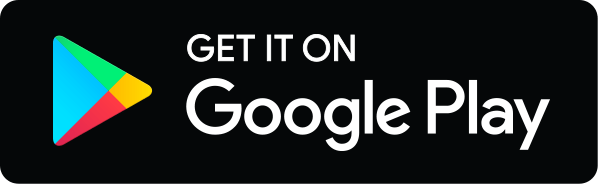