33 Clinical Validation: First-Generation Implants Indications and Contraindications Clinical Results of the Current Wallis Implant Dynamic intersegmental stabilization with the Wallis System (Abbott Spine, Inc., Austin, TX) implant lies within the framework of functional articular surgery. This implant, made of an interspinous process spacer that limits extension and two flexion-limiting bands, is intended to improve the stability of the treated intervertebral lumbar segment while preserving its mobility. The implant has an extra-articular design that makes it an easily reversible procedure. Because the operation leaves all the anatomical elements intact except for the interspinous ligament, the entire range of other surgical options remains open, including more invasive surgical solutions such as disk replacement or fusion. The primary goal of the Wallis System is to relieve or prevent low back pain that accompanies intervertebral segment instability. Furthermore, restoring a more physiological bio-mechanical environment to the degenerate lumbar segment with a Wallis may foster healing of the disk and facet joint lesions (provided that the disease process has not yet attained an overly advanced stage) and slow the degenerative cascade of adjacent segments. The purpose of this chapter is not to provide a full review of the origin and causes ofdegenerative disk disease (DDD), but rather to present the rationale and concepts upon which the Wallis System is based. The reader may find more detailed information on DDD elsewhere.1–4 Disk degeneration is a multifactorial process, the underlying causes of which remain poorly understood. Nevertheless, in all likelihood, DDD is mediated essentially by biomechanical, environmental, and genetic factors. In healthy intervertebral elements under normal physiological mechanical loads, balance between cellular anabolic and catabolic activity results in optimal remodeling of the nucleus pulposus extra-cellular matrix. The appropriate balance of disk cell activity results in stable, high proteoglycan concentrations that are necessary for the proper hydration and mechanical properties of the nucleus and annulus. This in turn ensures an adequate nutrient supply and removal of products of metabolism (lactate) as well as a beneficial cellular mechanical environment, further contributing to viable cellular activity and extracellular matrix synthesis and catabolism. Under such conditions, as long as the mechanical demands remain within physiological limits, this process of constant adaptive tissue remodeling maintains the functional anatomical architecture ofthe intervertebral disk (and ofthe facet joints as well). However, the balance between the external gravitational forces and internal forces may change due to either environmental conditions (work-related overloading) or age-related biomechanical changes (trunk deconditioning, insufficient muscular efficacy, or neuromuscular control). These abnormal conditions may disturb the equilibrium between anabolic and catabolic cellular activity (inadequate tissue remodeling) and result in a complex cascade of biological and biomechanical events that can alter the nucleus pulposus structure and ultimately lead to the overall disorganization and degeneration of the disk. Among other phenomena, this cascade involves either or both cell apoptosis and change in phenotype, which induce an inflammatory response with vascular invasion, macrophage infiltration, and macrophage/chondrocyte interaction. As a result, numerous proinflammatory cytokines, including interleukin (Il)-1a, Il-1b, Il-6, and tumor necrosis factor alpha (TNF-a) are produced in degenerative disks. The presence of these cytokines may further enhance the local inflammation and activate the production of enzymes (notably, matrix mettalo proteinases (MMPs) and aggrecanases) involved in the degradation of the extracellular matrix constituting a detrimental catabolic feedback loop. This degenerative process begins in the nucleus pulposus with cell loss and extracellular matrix alteration. Progression ofthe disease causes the outer annulus fibrosus (AF) to lose its normal lamellar arrangement and compromises the mechanical strength of the disk. Tears progressing from the inner AF outward contribute to the loss of mechanical integrity of the spinal segment. These changes increase the mechanical forces transferred to the surrounding vertebral end plates contributing to microfractures and marginal osteophyte formation. This altered mechanical environment could result in further and faster deterioration of the disk itself. This degeneration, which contributes to excessive mobility and abnormal loading, can transform the fibrocartilaginous architecture of the intervertebral disk into a pseudoarthrodial joint involving cell death in the nucleus pulposus and a cavitation phenomenon in the disk. Cell death rate also increases in the AF as well as in the cartilage of the vertebral end plates and facet joints. As already stated, susceptibility to this mechanically triggered cascade is widely variable depending on genetic factors, which consequently play a major role in the severity and rate of disk degeneration. Currently, the only available treatment modalities for disk-related spinal pain focus on alleviating symptoms rather than addressing the underlying cause of degeneration. It is likely that clinical outcomes for patients with painful intervertebral disk degeneration would improve if therapies were more focused on slowing, halting, or even reversing this process. One way of achieving this goal might be to artificially restore the physiological mechanical environment to alter the biomechanical parameters that sustain the catabolic feedback loop and disk destruction process. As early as 1986, we investigated this concept: rabbit studies performed in our unit showed evidence that disk lesions were reversible by artificially increasing resistance to compression and stretching of the segment using a ligamentoplasty that increased the intervertebral stiffness.5 These findings prompted us to design an implant that would reduce the range of motion (ROM) and increase the stiffness of a degenerative intervertebral segment. This approach was recently confirmed by Kroeber et al,6 who also concluded that degenerative changes could be reversed. Based upon a rabbit model of degenerative disk disease, their results suggested that regeneration of the disk can be induced by unloading of the intervertebral segment by dynamic axial distraction. The decompressed rabbit intervertebral disks showed signs of tissue recovery on biological, cellular, and biomechanical levels after 28 days of distraction. The implant consists ofan interspinous spacer that limits extension and two bands that secure the implant in the interspinous space and limit flexion. It was developed in two phases. In the initial system developed in the 1980s, the interspinous spacer was made of titanium. The spacer was connected to a woven polyester cord, which was looped around the spinous processes and reattached to the spacer by means of a morse taper. An improved Wallis device (Abbott Spine, Inc., Austin, TX) was developed in 20017(Figs. 33–1 and 33–2). The former metallic interspinous spacer was replaced by a redesigned spacer made of polyetheretherketone (PEEK). The initial woven polyester cord was replaced by bands made of the same material. Titanium crimping rings are added to prevent fraying of the extremities of the bands after cutting off the excess length. There is one radiodense tantalum marker in each side of the PEEK spacer. PEEK and polyester were chosen for their excellent biocompatibility, their radiolucency, and their lack of artifacts on magnetic resonance imaging (MRI). In addition, PEEK is highly resistant to degradation in a hydrous environment, and its modulus of elasticity is close to that of cortical bone, thus reducing the contact stresses between the spacer and the spinous processes in extension. The cord of the former device was replaced by flat bands to increase surface contact with the spinous processes, thereby minimizing local concentration ofcontact stresses on the bone during flexion. The implant also includes small titanium crimping rings and tantalum markers, which are radiodense and biocompatible and do not interfere with MRI. The PEEK spacer is designed for placement between the spinous processes of two adjacent vertebrae. There are two opposing grooves (proximal and distal) in the device to house the spinous processes. Each of these grooves is inclined 10 degrees with regard to the transverse plane for better adaptation to the anatomy of the spinous processes. The center of the spacer is completely traversed by two identical oval openings, and aligned with the grooves in the sagittal plane. These openings serve to further increase the flexibility of the spacer during compression loading of the intervertebral segment. Figure 33–1 The Wallis dynamic stabilization implant. Figure 33–2 A Wallis implant in place. At one corner of each side of the spacer, there is a tunnel loop around which is sewn one band extremity. After passage around the corresponding spinous process, each band is looped through a PEEK strap fastener, which in turn is snapped on the opposite side of the spacer into four notches located at the edges of a dedicated recess. Small retaining teeth in the floor of this recess prevent back sliding of the band. The implant position can be assessed by means of a radiodense marker (tantalum) in each lateral fastener. All spacers have the same width. Only the height increases with the size ofthe spacer. Wallis implants are available with interspinous heights of 8, 10, 12, 14, and 16 mm. All the materials in the device have a prior history ofimplant use. The biocompatibility of the PEEK and polyester materials themselves has been extensively tested and confirmed. Biocompatibility testing of the complete Wallis System was established for approval by the U.S. Food and Drug Administration (FDA) Investigational Device Exemption (IDE) study that is under way in the United States. The implant passed a battery ofstandardized tests, including cytotoxicity, irritation, sensitization, systemic toxicity, genotoxicity (Ames, chromosomal aberration, and mouse lymphoma assay [MLA]), pyrogenicity, and subchronic toxicity (implantation for 28 days, 3 months, and 6 months). Twelve randomly selected sterilized and packaged size 8 implants were submitted to static testing in saline (0.9%) at 37°C using a stainless steel model of spinous processes. The smallest implant (size 8) was chosen as the worst-case scenario because it has the weakest mechanical properties due to size. The static tension yield load (six implants) was 440 ± 14 N and the static compression yield load (six implants) was 1710 ± 107 N. These values can be put into perspective by comparing them to unpublished cadaver study values of the distractive force shared by the posterior arch during flexion (maximum value at bone failure: 130 N) and to published values of the resistance to compression of the spinous processes (mean value at bone failure, 339 N; 95% CI, 257–447 N).8 Under identical conditions (5 L of saline at 37°C and the stainless steel model ofspinous processes), six sterilized size 8 implants (worst-case scenario) were submitted to 10 million cycles at 5 Hz to test the compressive strength, and six other size 8 implants were submitted to 10 million cycles at 5 Hz to test the tensile strength. The dynamic run-out load was found to be 300 N in tension and 830 N in compression, both findings being more than twice the resistance values of posterior arches and spinous processes cited earlier. After 10 million cycles of tension at 300 N, the observed creep of the polyester band was 0.16 mm (0.5%). After 10 million cycles of compression at 830 N, the observed creep of the spacer was 0.04 mm (0.5%). Biomechanical evaluation of the effects of the new Wallis on spinal segment mobility and rigidity was performed in cadavers. Flexion-extension studies performed on intact and damaged disks (nucleotomy) demonstrated that the implant placed on a damaged structure restores the ROM to values similar to those obtained in healthy segments. In the altered segments, the implant also restored the stiffness values in a normal range. The stability of the segment was increased with reduction of the neutral zone and less translation. Although effective in stiffening flexion-extension movements, as intended, this interspinous dynamic system was shown not to affect either lateral bending or axial rotation of the instrumented segments. The effects of the new Wallis on the mechanical stresses applied to the disk were also investigated using a finite element model (FEM). The FEM demonstrated similar results in terms of spinal segment mobility when compared with the cadaver study (validating the FEM). Furthermore, the implant did not alter the mechanical behavior of the adjacent segment. In this FEM, implantation ofthe interspinous device reduced maximal compressive stresses within the disk, especially on its posterior aspect.
Dynamic Lumbar Stabilization with the
Wallis Interspinous Implant
Clinical Application of the Current Wallis System
Rationale for Use
Basic Concepts
The Wallis Implant
Overall Description
Preclinical Validations
Conventional Biocompatibility Testing
Static Tests
Dynamic Fatigue Tests
RUN-OUT VALUES IN TENSION AND COMPRESSION
CREEP AFTER 10 MILLION CYCLES
In Vitro Cadaver Testing
Finite Element Analysis
Clinical Validation: First-Generation Implants
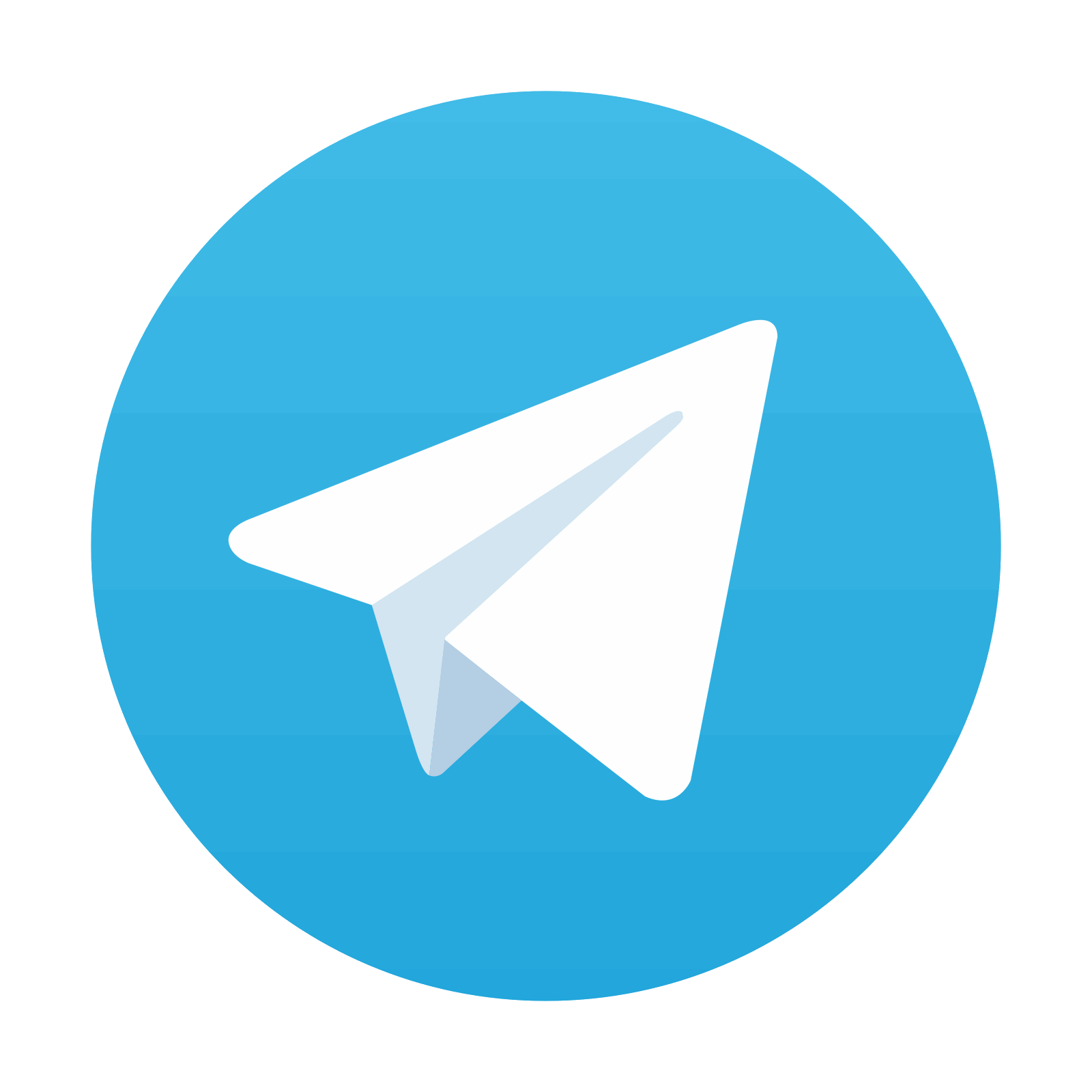
Stay updated, free articles. Join our Telegram channel

Full access? Get Clinical Tree
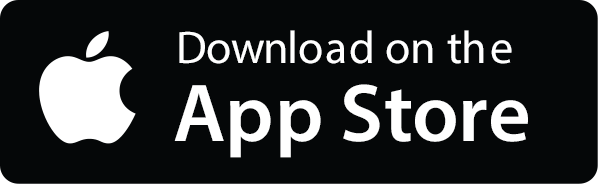
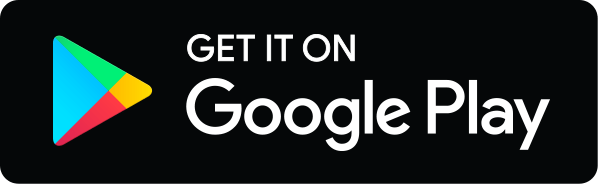