Imaging techniques capable of non-invasive, high-resolution, skin imaging in vivo have been the focus of recent attention in the dermatology field. These efforts are directed to improve the diagnostic accuracy of skin cancer, especially cutaneous melanoma.
Reflectance-mode confocal microscopy (RCM) allows real time non-invasive histological imaging with cytological detail comparable to conventional histology of the skin when exploring cutaneous structures between the stratum corneum and the upper reticular dermis.
Reflectance-mode confocal microscopes consist of a light source for illumination of a small spot within translucent tissue; and a point detector that detects back-scattered and reflected light though a pinhole. The pinhole prevents out-of-focus light from reaching the detector; as a result, only the optical plane in focus (confocal) is detected.
Similar to dermoscopy images, real time images obtained by RCM are oriented horizontal to skin surface (optical transversal sections).
Melanin provides strong contrast because of its high refractive index (1.7) relative to the surrounding epidermis; the melanosome size, similar to the near-infrared wavelengths (800–1,064 nm), produces strong back scattering of the beam. Thus, cells containing melanin, such as pigmented keratinocytes, melanocytes, or melanophages, appear very bright when illuminated in this manner.
Similarly, other organelles or cytoplasmic granules provide good contrast (albeit less intense than melanin), resulting in good imaging of cells containing them, such as Langerhans cells, lymphocytes, or cytoplasmic granules in keratinocytes at the stratum granulosum.
Major confocal imaging criteria of pigmented lesions such as benign and malignant melanocytic tumors have been established. RCM imaging criteria of other skin cancers such as basal cell carcinoma, squamous cell carcinoma, oral cavity neoplasm, and mycosis fungoides have also been evaluated.
Reflectance-mode confocal microscopy shows promise for: (1) guidance during biopsy collection; (2) monitoring histological architectural changes or dynamic process such as inflammatory response or capillary flow; (3) histological correlation with dermoscopic features; (4) monitoring of the response of a given lesion to treatment; and (5) demarcation of the extension of a lesion before proceeding to invasive treatments such as surgical excisions.
The main limitation of RCM is its relatively low penetration through the dermis; currently, a maximum depth of 250–300 μm can be achieved, preventing imaging of structures located in deep dermis and hypodermis.
The main challenge is the interpretation of images. Specific photographic atlas, courses and development of teledermatology may solve this problem.
Introduction
A wide variety of non-invasive imaging techniques provides the potential for high-resolution skin imaging in vivo including dermoscopy, optical coherence tomography, high-frequency ultrasound, magnetic resonance imaging, fluorescence-mode confocal microscopy, and reflectance-mode confocal microscopy. |
Reflectance-mode confocal microscopy is a technique with many possible applications, offering real time non-invasive microscopic images between stratum corneum and reticular dermis. |
Dermatology is an area of medical specialization in which diagnosis is frequently based exclusively on bare eyed clinical examination. Recent advances in imaging techniques provide the potential for non-invasive high-resolution skin imaging in vivo. These can overcome some of the disadvantages of the conventional biopsy and histologic analysis. Such advances include dermoscopy,1 optical coherence tomography,2 high-frequency ultrasound,3 magnetic resonance imaging (MRI),4 fluorescence-mode confocal microscopy,5 and reflectance-mode confocal microscopy (RCM). Of these, RCM is the technique with more potential applications, offering real time non-invasive microscopic images with the highest resolution comparable to routine histology when exploring cutaneous structures between stratum corneum and reticular dermis.6–8
History
Marvin Minsky invented confocal scanning microscopy in 1955. |
Development of light sources and computerization since the 1980s have significantly improved the hardware and software of confocal microscopy. |
Since the 1980s, several studies have demonstrated potential applications of in vivo RCM imaging in dermatology. |
The first paper using confocal scanning laser microscopy for imaging human skin in vivo was reported in 1995 by Rajadhyaksha et al.8 |
Marvin Minsky invented the confocal scanning microscope while working as a postdoctoral fellow at Harvard in 1955.9 However, its employment to image tissue in vivo required extensive development of light sources and computerization technologies. Since the 1980s, several research groups have employed tandem scanning confocal microscopy to image human and animal tissue in vivo.10–13 The first reported use of confocal scanning laser microscopy to image human skin in vivo dates back to 1995.8 Since then, many reports have suggested its possible applications in dermatology, particularly in the diagnosis of skin tumors.
Advantages of In Vivo Imaging in Dermatology
In vivo RCM imaging offers several important advantages over conventional histology: it is painless, causes no tissue damage and minimizes disruption of the native structure in the skin. |
The same region of the skin can be repeatedly imaged over time in order to evaluate tissue growth, wound healing, lesion progression or response to therapy. |
This technique collects data in real time and may be useful to study dynamic processes such as capillary flow or leukocyte trafficking. |
In vivo RCM offers several important advantages over conventional histology. Imaging is painless and non-invasive, causing no tissue damage. The skin is not altered by post-collection processing (fixation, sectioning, and mounting) or staining, minimizing artifacts or disruption of the native structure of the tissue. Real-time data collection is faster than routine histology, and the same location of the skin can be repeatedly imaged over time to evaluate dynamic changes such as tissue growth, wound healing, lesion progression or response to therapy.14–18 This is one of the main advantages of the technique compared to conventional histology, which can only reveal information about the tissue at the time of collection of the sample.
Principles of Reflectance-Mode Confocal Microscopy
Reflectance-mode confocal microscopy uses a point source of laser light (800–1,064 nm) that illuminates a small spot within translucent tissue such as skin or mucosa. |
The reflected light (reflectance) is imaged onto a detector after passing through a small pinhole. Backscattering of light occurs due to local variations of the refractive index within the tissue. |
The pinhole prevents out-of-focus light from reaching the detector in order to create a bi-dimensional image of a plane that is in focus (confocal) within tissue. |
The resolution depends on the pinhole size, the numerical aperture of the objective lens, and the wavelength used. Usually, RCM provides images with a lateral resolution of 1 μm and an axial resolution of 3–5 μm. |
The current depth that can be reached in the dermis with RCM varies among different microscopes (wavelengths), but averages 200–250 μm across. |
Water immersion lenses are used since the refractive index of water (1.33) is close to that of epidermis (1.34), minimizing spherical aberrations caused when the light passes through the tissue-air interface. |
A skin contact device is used to reduce motion artifacts and contain the water or gel interface during imaging. |
Melanin provides endogenous contrast because of its high refractive index relative to the surrounding epidermis and size of the melanosomes, similar to that of the illuminating wavelength. This means that cells containing melanin (pigmented keratinocytes, melanocytes, and melanophages) are bright under these conditions. |
By moving the lens along the “z” (vertical) axis with respect to the skin surface, it is possible to image different horizontal layers within the tissue. |
Images can be converted into static pictures of horizontal skin sections as well as recorded to produce movies of dynamics events, such as blood flow or trafficking leukocytes. |
Reflectance confocal microscopy is based on the use of a point source of light that illuminates a small spot within translucent tissue, such as skin or mucosa. The reflected light (reflectance) is imaged onto a detector after passing through a small pinhole. This pinhole prevents out-of-focus light from reaching the detector, so that only light from the exact in-focus plane (confocal) is detected. An entire image of the whole plane of the sample under study is generated using line scanning of the point source beam. This enables a virtual sectioning of a thin horizontal tissue plane in vivo, such as computerized tomography or MRI. Such optical sectioning of the tissue allows intact tissue to be studied without physical sectioning, as in conventional histology, preserving the physical structure and physiology of the tissue.
The resolution depends on the pinhole size, the numerical aperture of the objective lens, and the wavelength used. Although lasers of different wavelengths may be used as the light source for RCM, only longer wavelengths penetrate deep enough into the skin. Therefore, confocal microscopes use sources of illumination near the infrared wavelength (800–1,064 nm). Backscattering of light occurs due to local variations of the refractive index within the tissue as well as when the scattering structure has a size similar to the illuminating wavelength. For example, near-infrared wavelengths produce strong backscattering from melanosomes (despite melanin absorption at this wavelength) because they contain material with a high refractive index relative to the surrounding skin structures and have a size similar to the illuminating wavelength.14 This can be used in order to detect cells containing melanin, such as basal keratinocytes, melanocytes, and melanophages, which appear very bright upon imaging. Other microstructures similar in size to the illuminating wavelength, such as organelles or granules in the cytoplasm also act like reflectance surfaces and may determine other endogenous sources of contrast in Langerhans cells and lymphocytes.
New microscopes use a single optical fibre that is common to both the illumination optical path and the detection optical path. This single-moded optical fibre acts as a spatial filter that discards out-of-focus information collected by the objective lens. This system simplifies the opto-mechanical complexity by eliminating the bulky optical components and confocal apertures (pinholes) associated with conventional confocal implementations. This fiber-optic approach has enabled miniaturization of the confocal scanner into a hand-held device that provides the flexibility and mobility necessary for actual clinical use.
The currently commercially available RCM has a wavelength of 830 nm and 30× objective lens of NA 0.9, which provides a lateral resolution of approximately 1 μm and an axial resolution (section thickness) of 3–5 μm.14 It is possible to image normal skin from the stratum corneum to a depth of 200–250 μm, at the level of upper reticular dermis.14 Imaging deeper layers within the skin may also be achieved by using greater laser power, but the laser power used in the commercially available device is less than 30 mW because it causes no tissue damage or eye injury. Water immersion lenses are used since the refractive index of water (1.33) is close to that of the epidermis (1.34), which minimizes spherical aberrations caused when the light passes through the tissue-air interface.14 Other immersion media, such as water-based gels, are useful when imaging a scaly or hyperkeratotic lesion since the gel settles between disrupted corneocytes, reducing refractive irregularities. Such gels are also useful when imaging irregular skin sites, since the gel does not run off of the skin the way water or other aqueous solutions would do.
Skin movement is an important limitation when imaging in vivo. To reduce motion artefacts and contain the water or the gel interface when imaging, a skin contact device is used. This device consists of a metal ring that is fixed to the skin with adhesive, and is coupled to the microscope housing with a magnet during imaging. It has a concave shape to hold the immersion medium over a round window, which allows passing of light on to the sample as well as reflected light from the tissue.
Images are obtained after sequential illumination of multiple pixels according to the density of the reflected light from focal plane. This plane is scanned in two dimensions in order to create a mosaic image; architectural patterns can be evaluated this way. By moving the objective lens in the “z” stage (vertical) with respect to the skin surface, it is possible to image at different horizontal levels within the tissue since the focal plane is progressively moved deeper or closer to the surface. We can select each imaged square to compose a mosaic image and study cytological or architectural details. Images can be captured to produce static pictures of horizontal skin sections as well as recorded on videotape (20–30 frames per second) to produce movies that reveal dynamics events such as blood flow.8,14–18
Findings of Normal Skin
Confocal images obtained are horizontal or en face compared to vertical sections as normally obtained from routine histology. These images are grayscale (bright-scale) similar to radiographs or echographic examinations. |
The level of the skin under study can be ascertained by the morphologic appearance of tissue at a given depth or by measuring the depth of section, using a micrometer attached to the “z” stage of the objective lens. |
Starting from the surface and progressing deeper we elucidate the following tissue structures: |
Epidermis |
1. Stratum corneum. It appears as bright images of anucleated polygonal corneocytes 10–30 μm in size, and grouped in “islands” separated by skin folds, which appear very dark. |
2. Stratum granulosum. It has 2–4 layers of cells measuring 25–35 μm. The nuclei of these cells appear as dark central ovals, surrounded by bright grainy cytoplasms. |
3. Stratum spinosum. It has the aspect of a tight honeycomb pattern of smaller cells, measuring 15–25 μm in size, with well-demarcated cell borders. |
4. Basal layer. It consists of bright clusters of cells measuring 7–10 μm. The suprapapillary epidermal plate at the dermo-epidermal junction is apparent as rings of bright basal cells surrounding dark dermal papillae (bright dermal papillary rings). |
Dermis |
5. Papillary dermis. It consists of a network of reticulated gray fibers, with central capillary vessels surrounded by bright cellular rings correlated with basal keratinocytes. |
6. Reticular dermis. It shows a reticulated pattern, correlated with collagen bundles and elastic fibers. |
7. Skin appendages. |
Eccrine ducts appear as bright hollow structures that spiral through epidermis and dermis. |
Pilo-sebaceous units appear as whorled centrally hollow structures with elliptical elongated cells at the circumference and a central refractile long hair shaft. |
Although these are the classical features of normal skin, other variables such as anatomical site, sun-exposure, skin color, age, and physiological condition need to be analyzed. |
Real-time reflectance-mode confocal microscopy offers a novel view of the skin in terms of the orientation and image content. Compared to routine histology, it offers two main differences. First, the image obtained is horizontal or en face rather compared to the vertical sections typical in histology. Second, images are greyscale (bright scale), similar to radiographs or echographic examinations. The field of view provided by RCM varies with different microscopes, but is generally 250–500 μm across.14 The layer of the skin under study can be ascertained by the morphologic appearance of tissue at a given depth or by measuring the depth of section, using a micrometer attached to the “z” stage of the objective lens.
When imaging the skin, the most superficial images obtained are of the stratum corneum. The morphologic appearance of that section includes anucleated polygonal corneocytes 10–30 μm in size grouped in “islands” separated by skin folds, which appear very dark (Fig. 1a). Images are very bright because the refractive difference at the interface between the immersion medium and stratum corneum results in a large amount of back-scattered light. The next layer is the stratum granulosum, which consists of two to four layers of cells measuring 25–35 μm. The nuclei appear as dark central ovals within the cell surrounded by a bright grainy cytoplasm (Fig. 1b). The next layer is the stratum spinosum, which consists of a tight honeycomb pattern of smaller cells 15–25 μm in size with well-demarcated cell borders (Fig. 1c). The deepest layer of the epidermis is the basal layer, visualized as bright clusters of cells due to their content in melanin, 7–10 μm in size. The suprapapillary epidermal plate at the dermo-epidermal junction appears as rings of bright basal cells surrounding dark dermal papillae, which often show a central area of blood flow consistent with papillary dermal vascular loops (Fig. 1d). The papillary dermis images consist of a network of reticulated fibres with central capillary vessels (Fig. 1e). In the reticular dermis, confocal images show a thick reticulated pattern consisted of collagen bundles and elastic fibres (Fig. 1f). We can also observe eccrine ducts, which appear as bright, centrally hollow structures that spiral through epidermis and dermis, and hair shafts with pilo-sebaceous units. These appear as whorled, centrally hollow structures with elliptical, elongated cells at the circumference and a central, refractile long hair shaft.
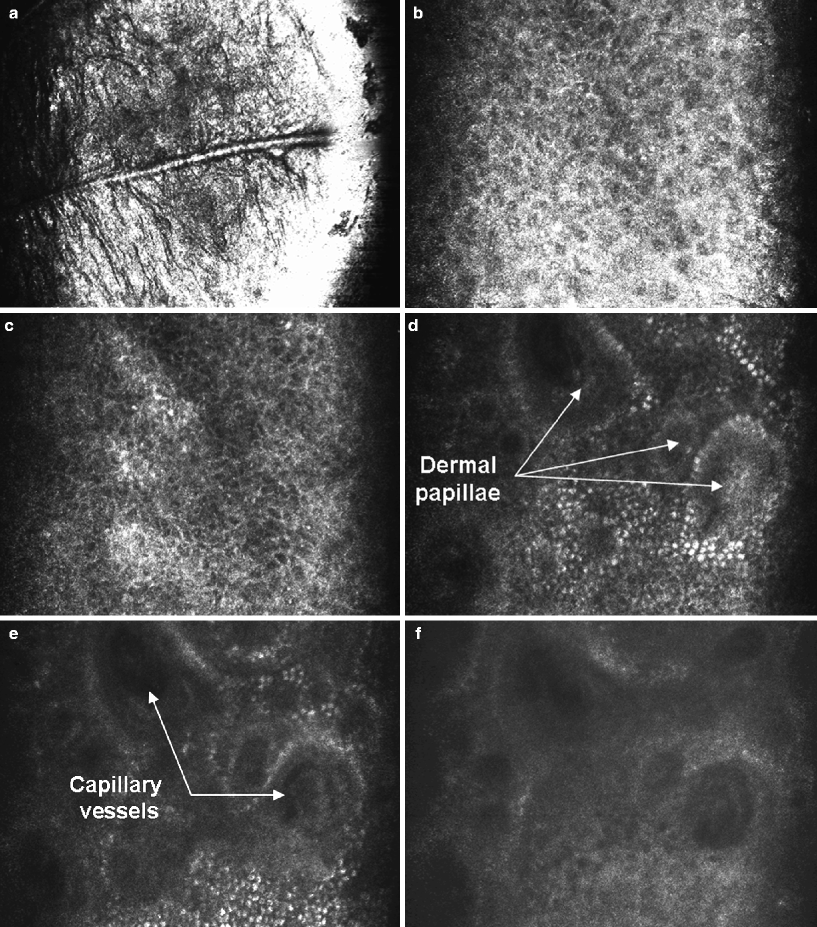
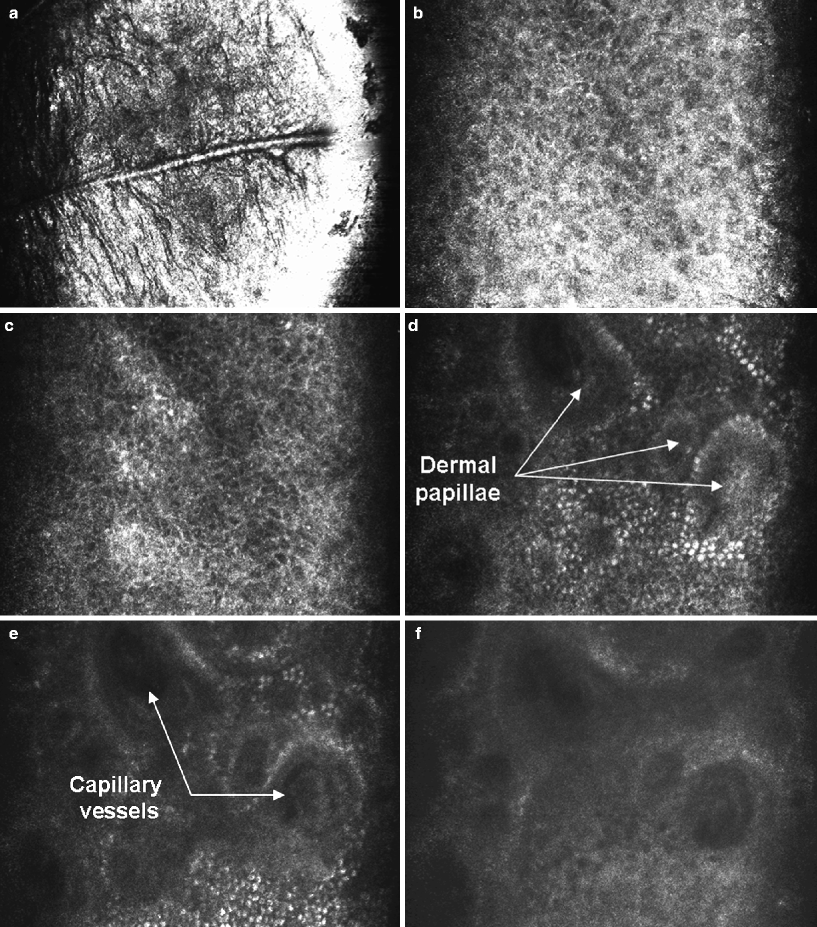
Fig. 1
RCM parallel images of normal skin obtained moving the objective lens in the “z” stage (vertical) with respect to the skin surface. Stratum corneum appears as a bright surface, with dark skin folds (a). The stratum granulosum has a regular honeycomb web pattern, where granular cells have dark oval areas (nuclei) within bright grainy cytoplasms (b). The stratum spinosum has a honeycombed pattern too, but spinous keratinocytes are smaller, with polygonal and well demarcated cell borders (c), The basal layer consists of small bright cells usually without nuclei visualized. They are located around of apertures of dermal papillae, which are delimited with uniform bright rings of basal cells (d–e). Dermal papillae have a thin network of reticulated grey fibres around dark spaces (capillary vessels), where blood flow can be visualized during real time imaging (e–f)
The appearance of normal skin varies according to the anatomical site, sun-exposure, skin color, age, and physiological condition being imaged.19 Skin from sun-exposed or darkly pigmented sites, for example, appears generally brighter because of what appears to be more pigment at the basal layer.
Non-Pigmented Lesions
Basal Cell Carcinoma (BCC) |
Confocal features of BCC have been defined retrospectively so that the presence of two of five criteria has a sensitivity of 100%, with a specificity of 95.7% when the number of criteria is four.21 |
Diagnostic criteria: |
1. Islands of monomorphic, elongated, tumor cells. |
2. Polarization of elongated, monomorphic nuclei along the same axis of orientation. |
Streaming: polarization of nuclei in an entire aggregate of tumor cells. |
Peripheral palisading of nuclei: peripheral monolayer of tumor cells oriented parallel to each other and perpendicular to the stroma. |
3. Variable epidermal disarray (nucleated corneocytes, loss of the honeycombed pattern, and keratinocytic nuclear pleomorphism). |
4. Prominent inflammatory cell infiltrate. |
5. Increase of capillary vessels, which are dilated and may show leukocytes rolling and their adhesion on endothelium. |
Actinic keratoses (AK) |
1. Irregular hyperkeratosis. |
2. Architectural disarray at the epidermal level: epidermal cell nuclear enlargement with pleomorphism and nucleated corneocytes (parakeratosis). |
3. Pattern of architectural disarray that does not involve the full thickness of the epidermis. |
The lack of depth of the illuminating wavelength restricts the potential of RCM to accurately differentiate between hypertrophic AK and SCC. |
Squamous cell carcinoma (SCC) |
1. Irregular hyperkeratosis. |
2. Architectural disarray at the epidermal level: epidermal cell nuclear enlargement with pleomorphism and nucleated corneocytes (parakeratosis). |
3. Pattern of architectural disarray involving the full thickness of epidermis. |
4. Vascular patterns and keratin pearls are not well characterized yet. |
The lack of depth of the illuminating wavelength restricts the potential of RCM to accurate distinguish AK from SCC, or between superficially invasive SCC and SCC in situ (Bowen’s disease). |
Oral cavity neoplasm |
1. Densely packed, pleomorphic tumor nuclei. |
2. Architectural disarray at the level of the epithelium with parakeratotic areas. |
3. Areas of inflammation. |
Mycosis fungoides (MF) |
1. Weakly refractile, round to oval point cells at epidermal levels (epidermotropic lymphocytes). |
2. Vesicle-like dark spaces filled with weakly refractile, round to oval point cells (Pautrier’s microabscesses). |
3. Increase of the intercellular space at epidermal levels; visualization of inter-keratinocytic junctions (spongiosis). |
4. Tagging of the dermo-epidermal junction by weakly bright, round to oval point cells (lymphocytic infiltration). |
5. Hyporefractility of the basal cells surrounding the dermal papillae. |
These confocal features are not specific since they have also been observed in lichenoid dermatitides and acute contact dermatitis. |
The characterization of skin neoplasm using RCM is an important area of clinical research with potential for non-invasive diagnosis and management of a variety of skin cancers. The advent of newer, less invasive topical therapies such as topical imiquimod or photodynamic therapy demands the development of non-invasive diagnostic tools to identify tumor subtypes and tumor margins accurately, and to monitor the response to treatment.
Basal Cell Carcinoma
Basal Cell Carcinomas (BCCs) are the most common skin tumors in humans (Fig. 2a, b); RCM features of BCC have been defined.20 They include the presence of islands of monomorphic tumor cells (Fig. 2c, d), elongated in shape and with nuclei oriented along the same axis, producing a polarized appearance (basaloid nuclei). This polarized cell pattern persists through the thickness of the epidermis (Fig. 2e), with loss of the normal progressive size difference of differentiating epidermal cells, loss of the normal honeycomb pattern, and loss of dermal papillae architecture. The presence of keratinocyte disarray and architectural disorder of the overlying epidermis is indicative of actinic damage or consequence of reactivity to the tumor. Reactive fibrosis is featured by thick, bright bundles near tumor islands (Fig. 2c, d). In addition, pronounced superficial blood vessels, dilation, tortuosity, and rolling of leukocytes along the endothelial wall have been frequently observed. It is also possible to visualize inflammatory cell infiltrates among the tumor cells (Fig. 2e).
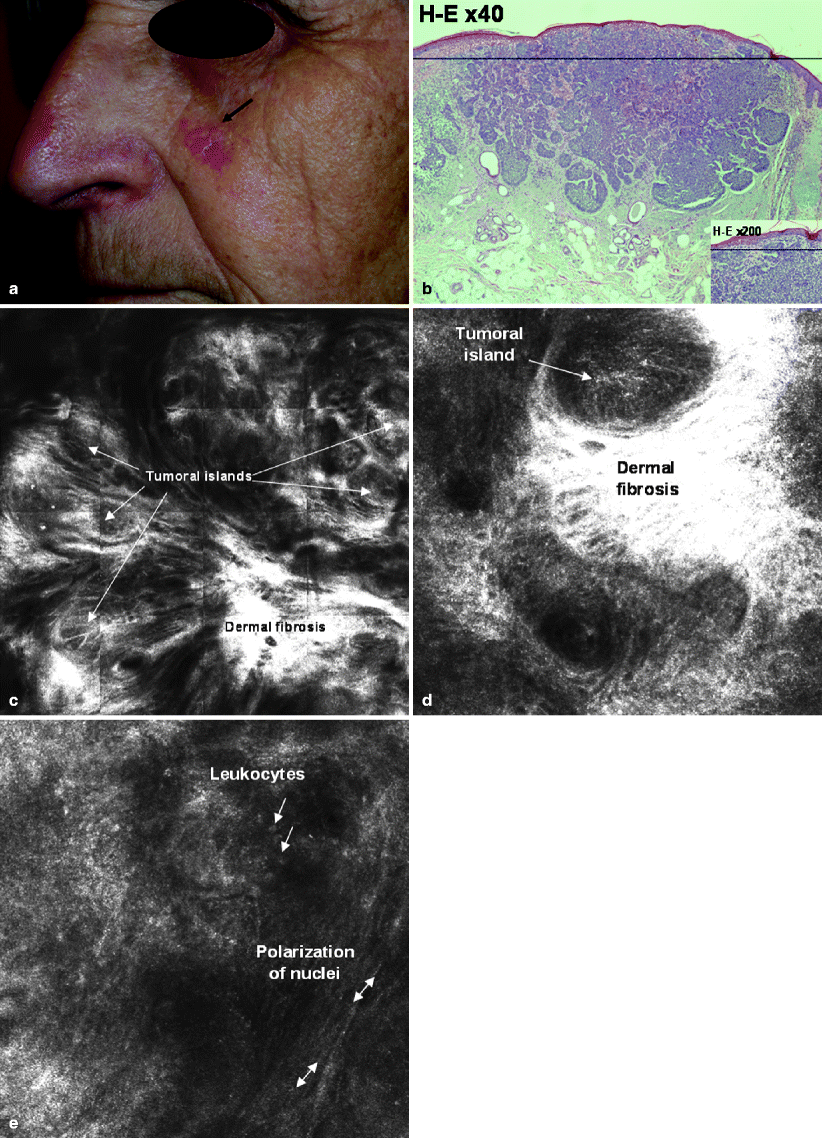
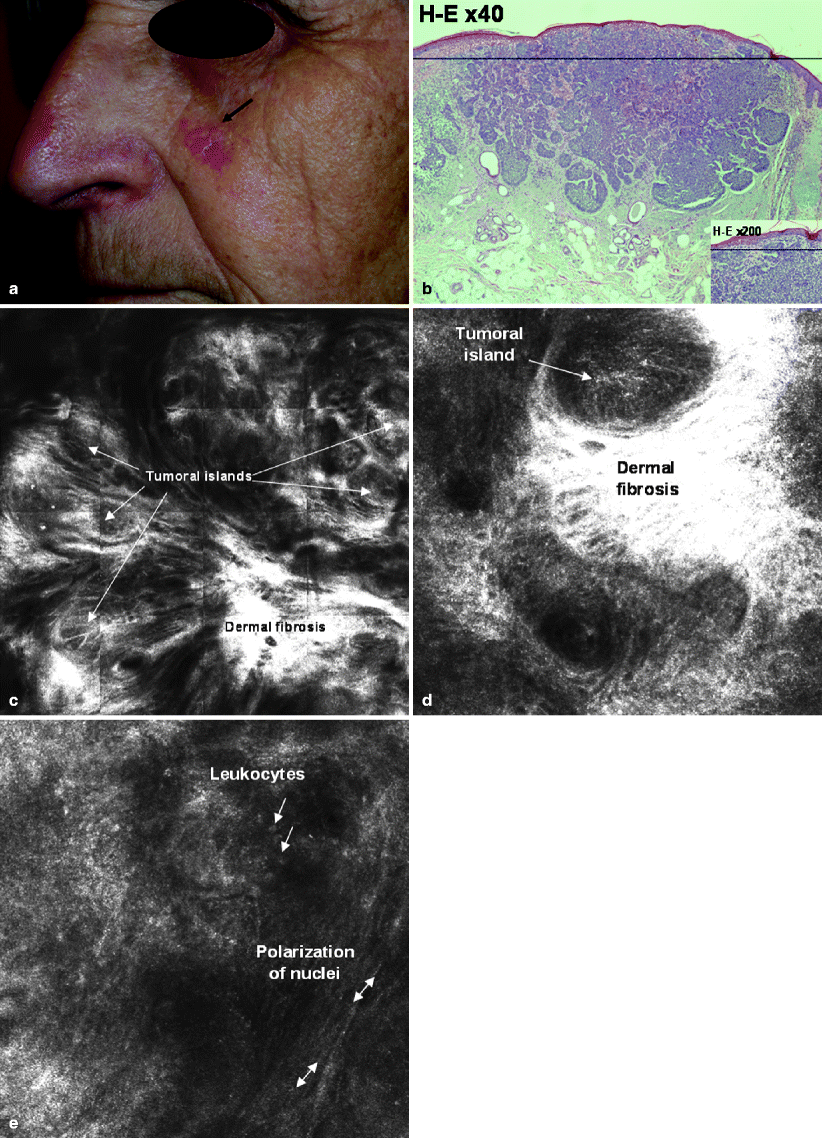
Fig. 2
(a) Clinical photograph of a BCC located on the left cheek of a 65 year old male showing superficial telangiectases and bright pearly borders. (b) H&E original magnification 40×, the corresponding histologic section shows tumor islands consisting of basaloid cells on a sun exposed (solar elastosis) skin. H&E original magnification 200×, corresponding histologic detail shows well-circumscribed tumor islands and their typical peripheral palisading. (c) RCM mosaic image taken at dermal level shows several tumor islands surrounded by thick gray collagen bundles. There are areas with brightly, dense, dermal fibrosis. (d) RCM image reveals loss of dermal papillae architecture and intense bright area, correlated with reactive dermal fibrosis, surrounding tumor islands (RCM image 500 × 500 μm). (e) RCM image shows a detail with long dark nuclei oriented along the same axis (polarized cell pattern) in a tumor island. Red arrows point to weakly bright cells among the tumor cells near the capillary vessels, which has been correlated with trafficking leukocytes and inflammatory cell infiltrate (RCM image 500 × 500 μm)
A retrospective, multicentric study from 152 lesions21 has determined that the presence of at least two of these criteria has a sensitivity of 100% for the diagnosis of BCC. As the number of criteria is higher, the specificity increased, so that when at least four criteria are present the specificity was 95.7%, showing the best correlation between high sensitivity and specificity. The most sensitive and specific criterion was the presence of polarized nuclei (91.6% and 97% respectively). These results showed little variability across BCC locations and subtypes. RCM may be also useful to noninvasively monitor the response to cryotherapy or new therapies such as topical imiquimod or photodynamic therapy.
Actinic Keratoses
Actinic keratoses (AKs) are keratinocytic dysplasias (Fig. 3a–c) that can develop into squamous cell carcinomas (SCC). Confocal features of AK include irregular hyperkeratosis with parakeratosis (Fig. 3d), architectural disarray, and epidermal cell nuclear enlargement with pleomorphism (Fig. 3e). The pattern of architectural disarray does not involve the full thickness of the epidermis.22 As BCCs, RCM images of AKs shows thick refractile bundles in dermis correlating with solar elastosis (Fig. 3f). The most important limiting factor of RCM is the shallow depth of the illuminating wavelength, which prevents accurate visualization of the dermo-epidermal junction, particularly in hyperkeratotic lesions. This restricts the potential of this tool to distinguish accurately between hypertrophic AK vs. SCC. On the other hand, recent evidence suggests the presence of multilocular preneoplastic changes in the areas surrounding the affected skin sites, thus RCM may be useful to detect subclinical AKs.23 The same report evaluated the sensitivity of RCM in the diagnosis of AKs. Sensitivity was an estimated 97.7% (44 AKs cases were included in this study). Thus, RCM may be a useful diagnostic tool in the management and follow-up of patients with low skin phototypes and a history of intense sun-exposure to permit an early diagnosis of AKs or BCCs.
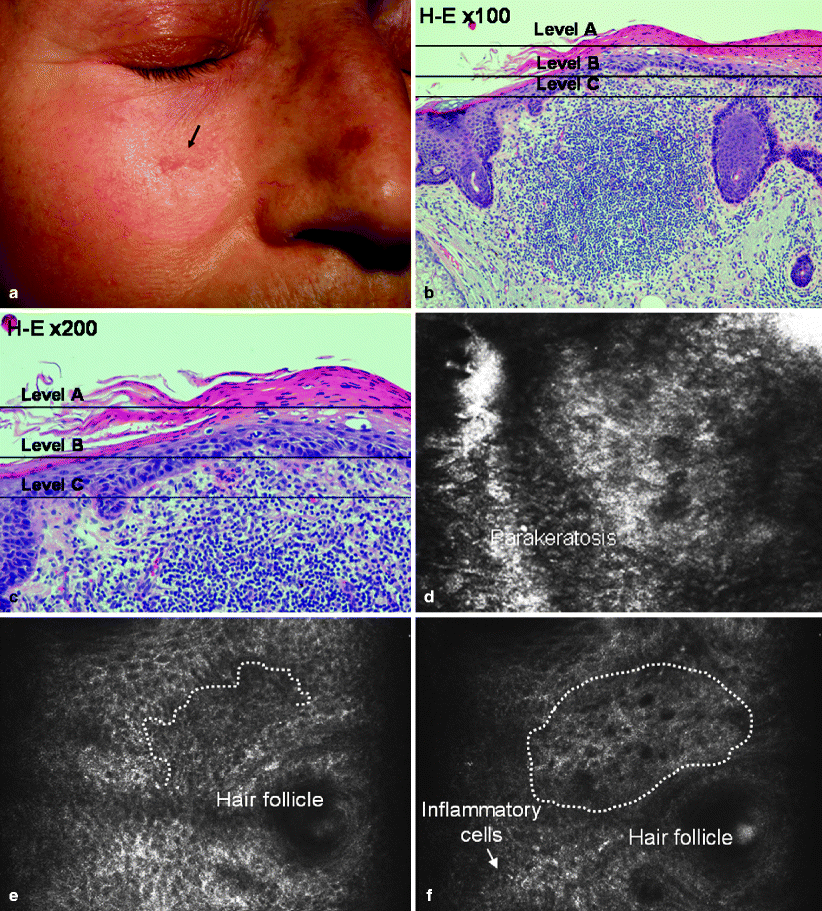
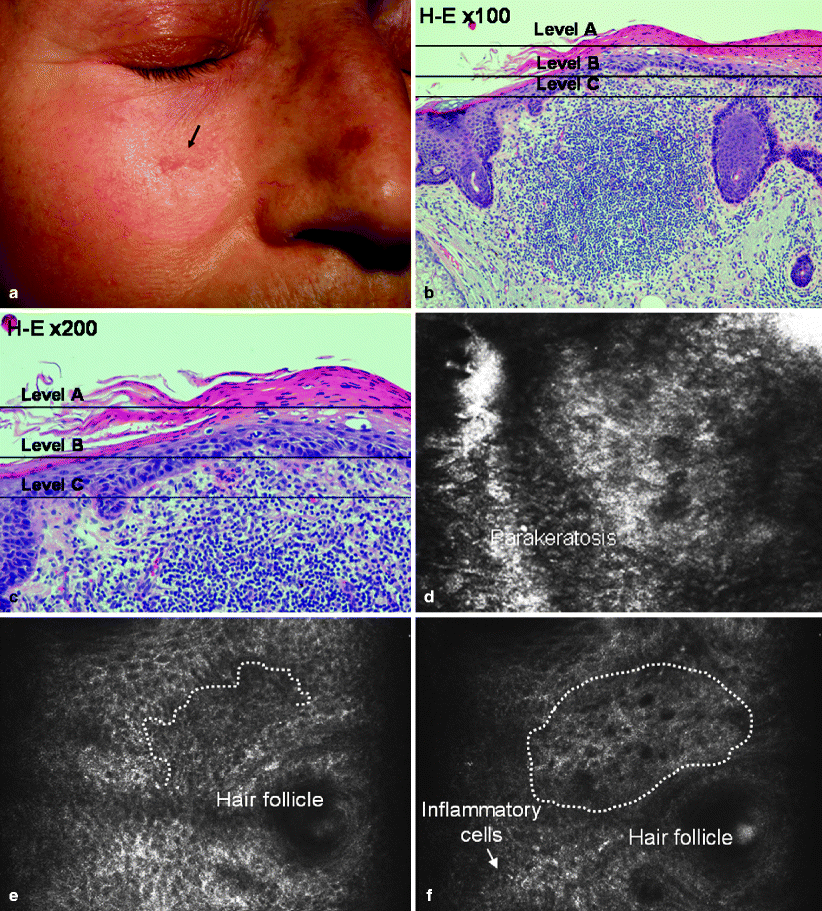
Fig. 3
(a) Clinical photograph of an actinic keratosis located on the right cheek of a 55 year old female. (b) H&E original magnification 100×, corresponding histologic section reveals parakeratosis and epidermal dysplasia involving interadnexal epidermis on sun exposed skin. These features support the diagnosis of actinic keratosis. (c) H&E original magnification 200×, corresponding histologic detail shows epidermal dysplasia affecting typically the basal layers, with parakeratosis and inflammatory infiltrates in dermis. (d–f) RCM images 500 × 500 μm at different levels of depth. Level A shows areas with bright polygonal and nucleated cells (parakeratosis) at the stratum corneum (RCM image 500 × 500 μm). Level B exhibits keratinocytic disarray and loss of honeycombed pattern. Dermal papilla (dotted line) is not well delimited showing the loss of uniform bright rings and distortion of DEJ limits (RCM 500 × 500 μm). Level C shows weakly bright point cells (inflammatory cells) and a thick gray network at the superficial dermis (dotted limited area) which has been related with actinic damage and correlated with solar elastosis (RCM image 500 × 500 μm)
Squamous Cell Carcinoma
Confocal features consistent with SCC are full thickness architectural disarray and nuclear enlargement with pleomorphism in the stratum granulosum and stratum spinosum22 (Fig. 4a–e). Other changes consistent with SCC, such as vascular patterns and keratin pearls, need further investigation. Leukocyte rolling along the endothelial wall has been observed as well (Fig. 4f). Lack of depth of the RCM illumination wavelengths makes differential diagnosis between hyperkeratotic AKs and SCC difficult due to lack of adequate visual assessment at the dermo-epidermal junction. This also makes differential diagnosis between superficially invasive SCC and SCC in situ (Bowen’s disease) impossible as of today.
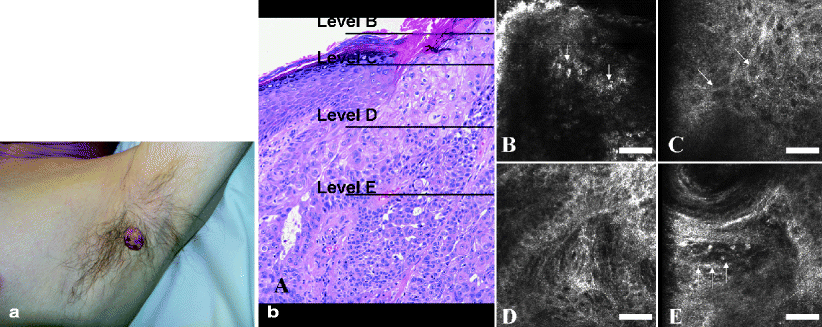
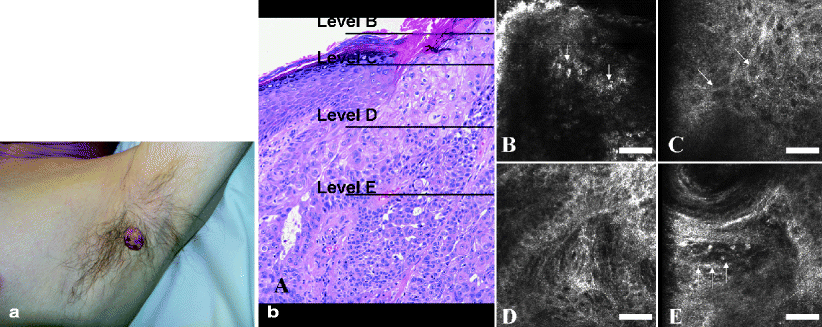
Fig. 4
(a) Clinical photograph of SCC located at the left axilla of a 57 year old male. (b) H&E original magnification 200×, corresponding skin biopsy exhibiting neoplastic cells with squamous differentiation grouped in tumoral nests within skin. (c–f) RCM images 500 × 500 μm at different levels of depth. Level B demonstrates nucleated corneocytes corresponding with parakeratotic cells (arrows). Level C shows keratinocytic disarray and the loss of honeycombed pattern at stratum spinosum. Level D exhibits several tumor islands and disruption of dermal papillae at DEJ level. Level E shows a RCM image taken at dermal level with dilated capillaries and weakly bright, point cells along the internal capillary surface (arrows) which have been correlated with rolling and trafficking leukocytes
Oral Cavity Neoplasm
Reflectance-mode confocal microscopy-based ex vivo examination allows imaging the oral mucosa with resolution comparable to conventional histology without tissue preparation and staining.24 In SCCs, densely packed, pleomorphic tumor nuclei, and architectural disarray at the level of epithelium could be visualized. Others features that could be identified included areas of inflammation. In vivo, imaging was possible up to 490 and 250 μm in the lip and tongue, respectively.25 Reflectance-mode confocal microscopy provides details of normal human oral mucosa at the cellular level; it has the potential for use in clinical practice as a diagnostic tool for the early detection of oral cancer and precancer.
Mycosis Fungoides
Reflectance-mode confocal microscopy is able to image epidermotropic lymphocytes, Pautrier’s microabscesses, spongiosis, and tagging of the dermo-epidermal junction by lymphocytes.26 Confocal features were hyporefractility of the basal cells surrounding the dermal papillae, corresponding to basal layer infiltration by atypical lymphocytes, and vesicle-like dark spaces filled with small, weakly refractile, round to oval cells, corresponding with Pautrier’s microabscesses. The latter was typically limited to plaque-type mycosis fungoides (MF). Hyporefractility of the basal cells surrounding the dermal papillae is a result of the presence of less refractile lymphocytes within and around the basal layer of the epidermis. Unfortunately, this hyporefractility has also been observed in lichenoid dermatitides and the vesicular structures may be difficult to distinguish from other vesicles filled with inflammatory cells, such as those seen in allergic contact dermatitis. Other limiting factor is the lack of depth, which is a key factor in tumoral-type MF. Reflectance-mode confocal microscopy may be a real-time guide for optimal biopsy site selection in patients with multiple lesions consistent with MF.
Pigmented Lesions
Pigmented Non-Melanocytic Lesions
Seborrheic keratosis |
1. Epidermal thickening without keratinocytic disarray. |
2. Long, parallel, dermal papillae. |
3. Homogeneous bright rings surrounding dermal papillae (edged papillae). |
4. Epidermal lesion with cerebriform architecture. |
Dermatofibroma (DF) |
1. Honeycomb pattern or cobblestone pattern at epidermal levels. |