22 The etiology of low back pain is widely variable and largely unknown. However, degenerative disk disease (DDD) can lead to disk dehydration, annular tears, and loss of disk height or collapse, and can result in abnormal motion of the segment and biomechanical instability causing pain.1 The diagnosis of DDD is determined as a result of radiographic diagnostic testing such as magnetic resonance imaging in conjunction with patient history and symptomatology. In patients suffering from intractable low back pain caused by DDD, nonoperative treatment including physical therapy, therapeutic injections, and management with analgesics often fails. In these cases, surgical intervention may be considered. Several techniques for lumbar fusion have been developed over the past 50 years that have become the standard of care for these patients, including rigid segmental pedicle screw fixation, titanium and polymer interbody cage fusion, and fusion involving preprocessed allograft interbody spacers. In addition to instrumentation and fusion devices, osteobiological materials have been developed to reduce or eliminate the need for iliac crest autograft while maintaining a high rate of solid arthrodesis. These include demineralized bone matrix, platelet-rich plasma, bone marrow aspirate, stem cell harvesting technology, bone morphogenetic proteins (BMPs), and numerous osteoconductive bone graft extenders. But while lumbar fusion serves to eliminate abnormal motion and instability at the symptomatic degenerated levels to reduce or eliminate low back pain for patients with DDD, reported clinical outcomes for these procedures vary widely. A recent meta-analysis performed by Geisler et al2 of clinical outcomes with minimum 2-year follow-up resulting from lumbar fusion procedures for the treatment of DDD showed the elimination of lumbar segmental motion resulted in a significant reduction in pain and subsequent improvement in overall function. However, as demonstrated in a cadaver study by Cunningham et al,3 lumbar fusion can lead to increased (abnormal) motion at levels similar to a lumbar fusion. Fusion is designed to eliminate the normal motion of one or more lumbar segments. Fusion is successful in many cases because the motion itself is the root cause of pain owing to the inability of the degenerative segment to support the weight of the body comfortably. Thus, when the segment is fused, it no longer moves and therefore cannot cause pain. Solid fusion, however, can result in stress and increased motion in the segments adjacent to the fused level,3,4 which may initiate or accelerate the degenerative disease process in adjacent segments. Hilibrand and colleagues5 demonstrated this concept in the cervical spine. The inherent problem with surgical arthrodesis of the degenerative lumbar segment is that it merely masks the true disease process by eliminating the intervertebral motion and its normal physiological function. In using lumbar artificial disk technology, the restoration and maintenance of normal physiological motion are provided rather than the alternative—the elimination of motion.6–9 The premise of surgery involving a lumbar artificial disk is fourfold: (1) correct abnormal motion; (2) restore intervertebral lumbar segmental space height, lordosis, and instantaneous axis of rotation; (3) maintain the corrected normal intervertebral motion over time; and (4) relieve pain and restore function. If these goals are achieved, it stands to reason that the segments adjacent to the dynamically stable segment would not be subject to abnormal loads and motions, and therefore, deceleration or elimination of adjacent level disk disease would follow. Numerous artificial disks have been designed during the past 35 years, but most have never been produced.10 There are four types of dynamic stabilization systems derived from artificial disk technology: (1) nucleus pulposus replacements with a hygroscopic gel or fluid-filled cylindrical sacs (for use after standard diskectomy in which the annulus maintains normal disk space height);11–13 (2) posterior dynamic stabilization systems (which increase posterior column stiffness);14–16 and (3) total lumbar joint replacement (which replaces both anterior and posterior lumbar motion segment components). Currently, these total joint replacement devices are not available in any U.S. Food and Drug Administration (FDA) trial, nor are any being used elsewhere in the world. Finally, total disk replacement is used to replace the entire lumbar disk. Because these devices replace only the disk portion of the joint, a partial joint replacement, they require healthy facet joints as well as intact posterior ligaments and muscular structures. An artificial lumbar disk should reproduce the biomechanical functions of a normal disk. Additionally, an artificial disk should reduce the mechanical forces transmitted to the adjacent levels, slowing or halting the degenerative changes. A total diskectomy eliminates the chance of a disk herniation and would probably retard spondylosis, stenosis, and instability at the dynamically stabilized segment. By restoring the disk space height, an artificial disk should restore normal motion, height, and lordosis; decrease the forces on the adjacent level (s); and prevent compression on the existing nerve roots at the stabilized level. The design of artificial lumbar disks has multiple, very strict requirements. These devices must possess superb mechanical strength and endurance. They are designed to last several decades because many will be implanted in young individuals. The base materials need to be biocompatible without causing significant surrounding inflammatory reaction either due to the base material reaction or secondary to any wear-related debris. The base material or potential debris of these devices must not produce organotoxic or carcinogenic reaction. The biomechanical functional movement requirements of an artificial lumbar disk are stringent because they need to replicate the full biomechanical functions of a normal disk. The normal motion of a lumbar segment includes independent translation and rotation in all three planes of motion (flexion-extension, lateral bending, and axial rotation). Normal motion is often represented as a factor of coupled motion in two planes. The implant-related geometrical configuration and materials would determine the static configuration, dynamic motion, schematics, and any constrained nature of the motion. The exact placement of the artificial lumbar disk in the disk space is determined by its biomechanical design. Different designs require different degrees of placement accuracy. Fixed pivot devices are believed to require a higher placement precision than devices that include a sliding core or an elastopolymer to decrease forces on the facets. Potential problems exist with regard to the choice of any base materials with which to construct an artificial disk. There is the possibility of the load-bearing surfaces becoming worn during the clinical lifetime of the device. Broadly, the materials are categorized into three groups: metal-on-metal, metal-ceramic, and metal-plastic designs. Those composed of metal-on-metal material have the potential of either or both metal and metal ionic debris. The ceramic component may shatter in metal/ceramic designs. Plastic wear or cold flow may occur in metal/plastic disks. The plastic components [cobalt-chromium-molybdenum (CoCrMo) and ultra high molecular weight polyethylene] in the current artificial hip and knee designs survive a mean of 10 years before requiring revision.17,18 If an artificial lumbar disk were made of these same base materials, it may be inferred that the plastic component would also require replacement at a mean of 10 years following implantation. There are, however, three primary differences between total joint replacement and artificial disk replacement. First, with each step the hip and the knee move ~50 degrees, whereas the lumbar spine only tilts a few degrees. This greatly decreases the so-called sandpaper effect by more than one order of magnitude for all base materials. Second, in the CHARITÉ Artificial Disc design (DePuy Spine, Raynham, MA), the high-density polyethylene moves to reduce the stresses, is not constrained, and has two opposite surfaces of contact that share the movement. This is in marked contrast to the hips, where the plastic is constrained in a high-pressure, ball and socket–type metal-plastic joint greatly accelerating wear on the plastic. The unconstrained sliding plastic core in the CHARITÉ lumbar disk has no pressure points. Furthermore, in a report from Europe, the authors noted the absence of plastic wear 10 years after implantation,19 which implies that the estimation of the lifetime of the material is greater than that used in the hips and knees. Four artificial disk designs have been the subject of U.S. FDA Investigational Device Exemption (IDE) trials (Fig. 22–1). It is notable that these devices do not repair the posterior column degenerative changes, nor do they augment them. In fact, a contraindication to application of any of these devices would be spondylolysis or significant spondylosis, with facet joint hypertrophy and potential for ongoing nerve root compression. These devices have either an unconstrained or a semiconstrained design with a fixed center of rotation. The original artificial ProDisc (Synthes, Inc., West Chester, PA) was designed by Thierry Marnay in the late 1980s. The current ProDisc design has a spherical articulation with two CoCrMo end plates and an ultra high molecular weight polyethylene core fixed to the lower device end plate, yielding a semiconstrained system. This design provides for a fixed pivot that places the instantaneous axis of rotation within the caudal vertebral body (VB) rather than the disk space. The ProDisc is affixed to the vertebral end plates by a central keel (or fin), which is driven into the vertebral end plates. Enrollment in the ProDisc IDE study has concluded, and the study is expected to be completed by the end of 2004 after a 2-year follow-up period. The FlexiCore artificial disk (Stryker Spine, Allendale, NJ) is a metal-on-metal semiconstrained device with a CoCrMo load-bearing surface with a 13 mm ball and socket joint, which places the stationary center of rotation centrally between the end plates. It has teeth on the outer ring of the implant end plates for fixation to the outer ring of the vertebral end plates. The U.S. FDA IDE trial of the FlexiCore artificial disk began in August 2003. The Maverick artificial disk (Medtronic Sofamor Danek, Memphis, TN) has a semiconstrained metal-on-metal design. Like the ProDisc, the Maverick implant has central keels that are driven into the vertebral end plates for fixation and stability. Enrollment in the Maverick IDE trial has concluded and FDA approval is expected in 2006. Figure 22–1 The four lumbar artificial disks in or finished with U.S. Food and Drug Administration I Investigational Device Exemption clinical trials. The CHARITÉ Artificial Disc (Fig. 22–2A–D) was designed to duplicate the kinematics and dynamics of a normal lumbar motion segment10,20 while restoring disk space height and motion segment flexibility. The CHARITÉ Artificial Disc is composed of two CoCrMo end plates and a free-floating ultra high molecular weight polyethylene core. The primary attachment of the plates is made possible by three anterior and posterior “teeth,” which are forcefully implanted into the cranial and caudal vertebral end plates. Layers of plasma-sprayed porous titanium and calcium phosphate were added to the Charité disk in 1998. This coating provides for potential osseous ingrowth and long-term stability of the plates after implantation.21 The plates are currently available in seven footprint geometrical configurations (including three wide footprints) adaptable to the size of the vertebral end plates, each with four available angles (0,5,7.5, and 10 degrees). This allows for built-in lordosis with variations of 0 to 20 degrees. The optimal device placement is 2 mm dorsal of the sagittal vertebral body midline and in the midline from right-left perspective with the metal end plates on the circumferential cortical bone (Fig. 22–3A,B). The unconstrained design allows the core to translate dynamically within the disk space during normal spinal motion, moving posteriorly in flexion and anteriorly in lumbar extension. The mobile sliding core of the Charité disk works in a similar fashion to the mobile knee bearing in many of the contemporary knee implant designs. In essence, this could be considered a second-generation device or an advanced type design over a fixed pivot, much like the mobile core in the knee is considered an advanced design over fixed bearings. The Charité design not only provides unloading of the posterior facet structures during this normal replication of motion but also allows forgiveness for slight off-center positioning of the implant. In a cadaveric model, Cunningham and associates3 demonstrated that the center of rotation for Charité closely mimicked that of a normal lumbar disk at the level of implantation and at the superior adjacent level. Fusion, however, greatly distorted the center of rotation at the level of implantation and at the superior adjacent level. In addition, compared with a normal intact segment, the Charité device did not adversely affect the range of motion (ROM) at adjacent levels, whereas fusion caused a “marked increase” in adjacent level motion. The coupled translation with angulation was noted in the normal spine and reproduced at the level with the CHARITÉ Artificial Disc (Fig. 22–4A,B). He further noted that the ROM was increased at the adjacent levels fused, in marked contrast to the placement of the CHARITÉ Artificial Disc, which preserved motion at both the level operated and the adjacent levels (Fig. 22–4C). This increased range of motion and hence forces and stress at the adjacent level is hypothesized to be a major contributing factor to accelerating adjacent level degenerative changes. The cortical rim of the vertebral bone is mechanically stronger than the central cancellous portion of the vertebral body. To lessen the chance for subsidence of the body into the bony end plate the largest CHARITÉ metal end plate should be utilized (Fig. 22–5A–C). Figure 22–2 (A) CHARITÉ Artificial Disc in an assembled form. (B) The components of the CHARITÉ Artificial Disc are available in multiple end plate sizes and core heights with four lordotic end plate styles allowing for restoration of lordosis from 0 to 20 degrees. (C) The translation provided by the mobile core of the CHARITÉ Artificial Disc, also shown on (D) plain flexion-extension radiographs. Figure 22–3 To match the location of the floating center of rotation of a normal lumbar disk, the CHARITÉ Artificial Disc should be placed (A)2 mm dorsal to the sagittal midline in the lateral view and (B) in the midline in the anteroposterior view. Finite element analysis supports this concept. In a two-level, three-dimensional, nonlinear, finite element model, Moumene and Geisler22 described the effect of fusion compared with CHARITÉ disk–related treatment on the facet loading of the adjacent segment. In axial rotation, fusion increased the load on the facet joints at the adjacent level by 96% compared with the normal intact nonoperated segment. The CHARITÉ device decreased the facet joint load at the adjacent level by 50% compared with the normal intact nonoperated segment (Fig. 22–6A,B). The CHARITÉ Artificial Disc has been used outside the United States since 1987, and in the United States since 2000. Worldwide experience with this unconstrained anatomical replacement disk now comprises more than 11,000 cases. Cinotti and colleagues23
CHARITÉ Artificial Disc
Rationale for an Artificial Disk
Clinical History of the CHARITÉ Artificial Disc
The FDA IDE Multicenter Trial of the CHARITÉ Artificial Disc
Rationale for an Artificial Disk
History
The CHARITÉ Artificial Disc
Clinical History of the CHARITÉ Artificial Disc
Stay updated, free articles. Join our Telegram channel

Full access? Get Clinical Tree
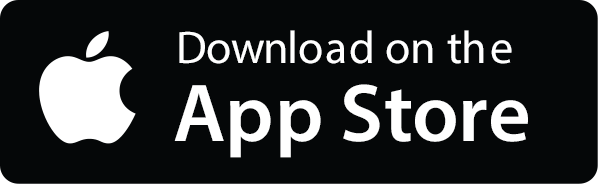
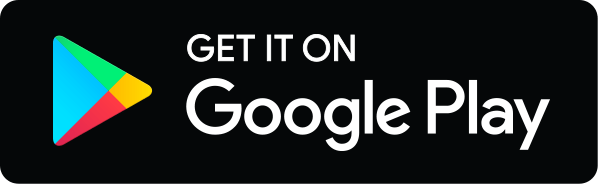