CHAPTER 62 Bearing Surface
Metal on Metal
TRIBOLOGY
The three pillars of tribology—friction, lubrication, and wear—are inevitable consequences of allowing load to be transmitted in vivo through two interacting surfaces in relative motion. Cobalt-based alloys are used exclusively for MM hip implants, and the MM pairing is used exclusively in hip resurfacing arthroplasty (Fig. 62-1). The principle constituents of these alloys are cobalt, chromium, and molybdenum, but they can be differentiated as being either high carbon (0.20% to 0.25%) or low carbon (0.05% to 0.08%), with the former usually demonstrating lower in vitro wear rates.1,2 MM hip bearings produce wear particles by some combination of the four classic mechanisms of adhesion, abrasion, corrosion, and surface fatigue, with an emphasis on abrasion and surface fatigue.3 The in vivo volumetric wear has been estimated from implant retrieval studies,4,5 and it increases with implantation time. The type of lubrication influences the severity of friction and wear. In simulator studies, the steady-state wear rate and the amount of running-in wear have been related to elastohydrodynamic film thickness for mean operating conditions of load, speed, and viscosity, along with elastic properties and combined surface roughness of the metals employed in each prosthesis.6–9 Using the data of other investigators, Paré and colleagues4 have suggested that in vivo run-in wear is higher than the steady-state wear that is established after about 18 months, providing dislocation and loosening do not occur.
Recent concerns with MM hip bearings are related to wear, including the elevated metal ion levels in the blood of patients, mostly caused by in situ corrosion of the wear particles, as well as the possibility of biologic responses to these ions and wear particles.10 There is further concern regarding fixation issues that are related to load and friction,11–16 alloy metallurgy,17 and elevated wear from microseparation.18 The purpose of this section is to identify the essential features of MM hip bearings that significantly influence tribologic performance and to suggest strategies regarding implant selection.
Lubrication and Geometry
Ultimately the clinical performance must provide the proof that an arthroplasty procedure is effective and that the implant involved is optimal. However, implant performance can be examined using wear simulators, with some measure of reliability and long before the clinical performance is ascertained. It is important to remember, however, that a wear simulator evaluates the hip bearing under conditions that represent some aspects of the clinical situation, and therefore a wear simulator does not necessarily provide good fidelity with all clinical situations. In particular, the details of the surgery including implant alignment, ligament tensions, and fixation are not usually included in the simulation. However, microseparation damage, which is presumably related to ligament tensions, has been examined in simulator testing18 and has been shown to increase wear significantly.
Having qualified the expectations of wear simulators, they do remain an important investigative approach. For example, the simulator wear of MM hip implants has been shown by Chan and colleagues6 and later by Paré and colleagues4 to decrease with an increasing ratio of the theoretical elastohydrodynamic lubricant film thickness to the measured initial combined root mean square surface roughness, known as the lambda (λ) ratio. Such behavior is consistent with the usual tribologic interpretation of the lambda ratio. For λ ≤ 1, boundary lubrication involving the protection afforded by lubricant molecules that have weak chemical attachments to the surface tends to dominate, whereas for λ ≥ 3, fluid film lubrication tends to dominate with a physical entrainment of a fluid between the surfaces that depends on fluid rheological (or flow) properties. For MM hip implants (and other applications) the fluid film lubrication also depends on surface deformation and thus is called elastohydrodynamic lubrication. In simulator testing, the fluid film lubrication regimen (λ ≥ 3) has been more effective than boundary lubrication in reducing wear.4,6
To link this fluid film lubrication behavior to clinical reality, a simple geometric quantity for the contact, known as the effective radius (R), was specified by Medley and colleagues19 as R = RH (RH + C)/C ≅ RH2/C where C = RC − RH, RH = radius of curvature of the head, and RC = radius of curvature of the cup. The effective radius combined the size of the implant (RH) and the radial clearance (C) into a single parameter. Because λ increased in an almost direct proportion with R, it was possible to correlate the steady-state volumetric wear rate (VR in cubic millimeters per year) both in simulators and as determined from retrieved implants with the effective radius (Fig. 62-2), where a value of 2 million cycles (Mc) in the simulator was assumed to be equivalent to 1 year in vivo. Dowson23 presented correlations of similar scope to those in Figure 62-2 but involving the relationship of both run-in and steady-state simulator wear with theoretical lubricant film thickness. The present study simplified this approach (by not differentiating between run-in and steady-state wear and by correlating wear with R and not theoretical film thickness) but still had a somewhat similar rationale.
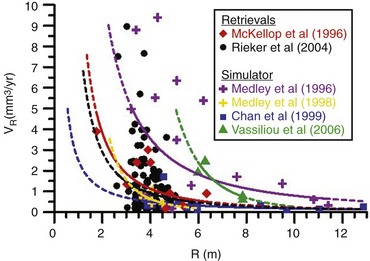
(Atypical data points removed: three from McKellop and colleagues,20 one from Vassiliou and colleagues,21 one from Chan and colleagues,6 three from Medley and colleagues,7 and seven from Rieker and colleagues.22)
In Figure 62-2, the wear was assumed to be 1.5 times the wear of the head for the data of McKellop and colleagues,20 who had measured the volumetric wear of the heads (VH) of mostly McKee-Farrar implants of 34.9 to 41.3 mm in diameter that had been in vivo for 1 to 24 years. Rieker and colleagues22 measured linear wear (sum of maximum depth of wear penetration in both head and cup) in mostly 28-mm–diameter implants that had been in vivo for up to 10 years. In order to include the data of Rieker and colleagues in Figure 62-2, linear wear (L) in micrometers was converted to volumetric wear (V) in cubic millimeters using the formula V = 0.0466L1.47 (Fig. 62-3), which was derived from the following:

Then V was divided by implantation time to get the volumetric wear rate (VR).
Data values from wear simulators were also included in Figure 62-2. Using a MATCO hip simulator, Medley and colleagues7,24 measured simulator wear with progressively improved precision, and eventually Chan and colleagues6 measured simulator wear of 28-mm–diameter implants with high R values; data from all of these studies were included in Figure 62-2. In addition, simulator data from Vassiliou and colleagues21 was included. They measured the wear of 50-mm–diameter Birmingham Hip Resurfacing implants (supplied by Midland Medical Technologies [Smith and Nephew], UK), in a Durham hip simulator for 5 Mc.
Effective radius (R) is not the only factor involved in lubricant film generation and wear reduction, but, as shown in Figure 62-2, it is a powerful one, for both simulator wear rates and estimated in vivo wear rates. The R value can be optimized for design purposes, especially with hip resurfacing implants.26 A high R value ensures that lubricant can be entrained into a gradually convergent gap between the surfaces, and it ensures that when there is direct contact the normal stresses remain relatively low.13,14,19 Implant components can be surface ground to specific radii of curvature with little waviness to produce low radial clearances and thus high R. The clearance must not be too low because a gap in the equatorial region is needed to avoid a combination of lubricant starvation and direct surface contact that can lead to high frictional torque15 and implant seizure, causing extensive surface damage and loss of fixation. Cup deformation also has to be considered when one decides how low a clearance to design.12,14,16 However, assuming seizure can be avoided, a low clearance and thus high effective radius can usually give thicker lubricant films and, when direct contact does occur, lower contact stress. Low levels of waviness are also likely to be important in promoting thick films and low contact stress. The R value can be set by the manufacturer and remains relatively constant in vivo and therefore is one of the most useful ways to control wear in MM hip implants. A beneficial larger R can be achieved by a large head and as low a clearance as permitted by manufacturing tolerances.
If large-diameter, low-clearance precision ground implants are used, Figure 62-2 suggests that volumetric wear rates can be driven to virtually zero, thus reducing most wear-related problems. Furthermore, subtle changes in surface geometry may help maintain beneficial lubrication effects in the long-term performance of MM hip implants.13 However, as extra insurance—for instance, for highly active patients27 or in the event of microseparation damage18—the addition of a protective surface layer may be very beneficial if it remains intact over the long term, especially in reducing blood ion levels.11,18
Alloy Microstructure
Lubrication and geometry, even with the help of a protective surface coating, cannot solve all MM wear problems because some direct contact must occur during stop-dwell-start motions in vivo.4 Under conditions of direct contact, boundary lubrication and surface microstructure become important in influencing wear. The boundary lubrication is not very well understood28 and is difficult to influence in any case. The influence of surface microstructure is under the control of the manufacturer, but the relationship to long-term wear is not well understood either. It is important to mention that MM bearings can be manufactured as wrought or cast materials. Although these two materials display similar chemical compositions, their microstructures are quite different. The wrought material has a small grain size with a fine homogenous distribution of small carbides, whereas the cast material exhibits large grains with blocky carbides. In order to produce more uniformly distributed carbides along grain boundaries, some implant manufacturers submit their implants to a high-temperature heat treatment process.1
Wear studies for cobalt-based alloys (used exclusively for MM hip implants) have been conducted in a 25% serum solution with a linear-tracking, reciprocating pin on plate apparatus to examine the influence of microstructure on wear. Varano and colleagues2 found that dissolved carbon (in solid solution, not carbide form) was effective in stabilizing the face-centered-cubic phase of the cobalt-based alloy and thus inhibited strain-induced transformation (SIT) to the hexagonal close-packed phase to give lower wear (Fig. 62-4). Varano and colleagues2 also found a somewhat lower wear level for as-cast compared with solution-annealed cast cobalt-based alloy (a topic of some industrial and commercial interest). This finding was probably related to the performance in the pin on plate apparatus of the different carbide morphologies and might not be clinically significant because the simulator studies of Bowsher and colleagues27 did not find this material-processing effect. However, McMinn and Daniel29 remained convinced that solution annealing can result in elevated wear and clinical problems in hip resurfacing arthroplasty.
Concluding Remarks on the Tribology
Taken together, the results presented (see Figs. 62-2 and 62-4) suggest that an implant with large effective radius (R) and high dissolved carbon would benefit from improved lubrication and reduced SIT to achieve lower wear. The lower contact stress associated with the large effective radius would further discourage SIT. The effective radius and dissolved carbon content are both parameters that are under manufacturers’ control and remain relatively constant in vivo. Therefore a simple yet potentially effective strategy for manufacturers has been identified, and some key tribologic issues discussed along the way. Recently, Medley has provided a detailed account of MM hip implant tribology.30
Specifically, with good manufacturing precision a larger-diameter implant provides an opportunity to achieve a high R value and thus lower wear. Surface microstructure may be important in reducing long-term wear, and higher levels of dissolved carbon may be beneficial. The efforts to introduce a protective surface layer may be very effective in reducing wear.11,18 Problems may still exist with frictional torque and long-term fixation, but the revision rates reported by Rieker and colleagues22 are not alarming.
CLINICAL PERFORMANCE
Although MM total hips started to be implanted around the same time as low frictional torque MPE prostheses, early failures of poorly designed MM total hips such as the Stanmore contributed to the popularity of the Charnley concept of the MPE bearing. However, long-term follow-up looking at other implants such as the McKee-Farrar showed a cumulative survivorship at 20 years of 77% compared with 73% for the Charnley implants.31 These results, combined with their own observations of the good performance of the McKee-Farrar prostheses, motivated Müller and Weber to re-explore MM bearings in 1984.22,32 With the assistance of Sulzer Orthopaedics (now part of Zimmer), they developed the Metasul bearing, which is a MM hip implant made from wrought cobalt chromium molybdenum alloy with high carbon content (0.20% to 0.30%).22
Although many of the initial designs were all-cemented implants,32 long-term results with total hip arthroplasty (THA) and even more so with hip resurfacing showed a high incidence of radiolucencies33,34 and a high failure rate of the cemented acetabular component, respectively, leading the majority of implant manufacturers to opt for cementless acetabular fixation.35–38 Currently it is estimated that more than 300,000 of these second- or modern-generation components have been implanted in patients worldwide, with cementless and/or hybrid designs showing excellent survivorship for both hip resurfacing and stem-type hip replacements.34–42
In terms of actual in vivo wear, early data come from retrieved McKee-Farrar prostheses, which had an average linear wear rate of 0.003 mm/year and 0.004 mm/year for the femoral head and cup, respectively.20 It is interesting to note that the larger-diameter femoral heads (42 mm versus 35 mm) had a twofold lower mean volumetric wear rate, 0.7 mm35 and 1.4 mm35 per year.20 In a key article, Rieker and colleagues22 reported on the largest retrieval analysis of failed MM bearings of the current generation (high carbon, wrought cobalt chromium molybdenum alloy). They analyzed 608 individual retrievals (femoral heads and/or cups from 337 revisions), with the vast majority of implants having a diameter of 28 mm. The linear wear rate for the first year in vivo averaged 27.8 µm/year and was mainly influenced by the prostheses that were revised because of dislocation. The wear rate after the second year in vivo averaged 6.2 µm/year, with femoral heads generally exhibiting a higher wear rate compared with the cups. There was also a positive correlation between clearance and wear rate. In contrast, Reinisch and colleagues43 reporting on the retrieval of a low carbon cobalt chromium alloy found a higher mean linear wear rate of 7.6 µm/year, even though the bearing had the same diameter as, and clearances comparable to, those reported by Rieker and colleagues.
Metal-on-Metal Hip Resurfacing
Hip resurfacing is no longer at the stage of evaluation and is regularly being offered as an alternative to THA for the young and active adult.44,45 As in other forms of conservative hip surgery,46 patient selection has helped minimize complications39 and risk of failure.41 The reintroduction of hip resurfacing as an MM bearing went through a variety of designs including all cemented as well as all cementless designs. Both short-term34 and long-term results have shown a poor survivorship of cemented acetabular fixation, with Beaule and colleagues33 reporting 66% at 7 years. Currently, hybrid fixation has the greatest amount of clinical data, with four major clinical series reporting survivorships of 97% to 99% at 4 to 5 years.39,41,
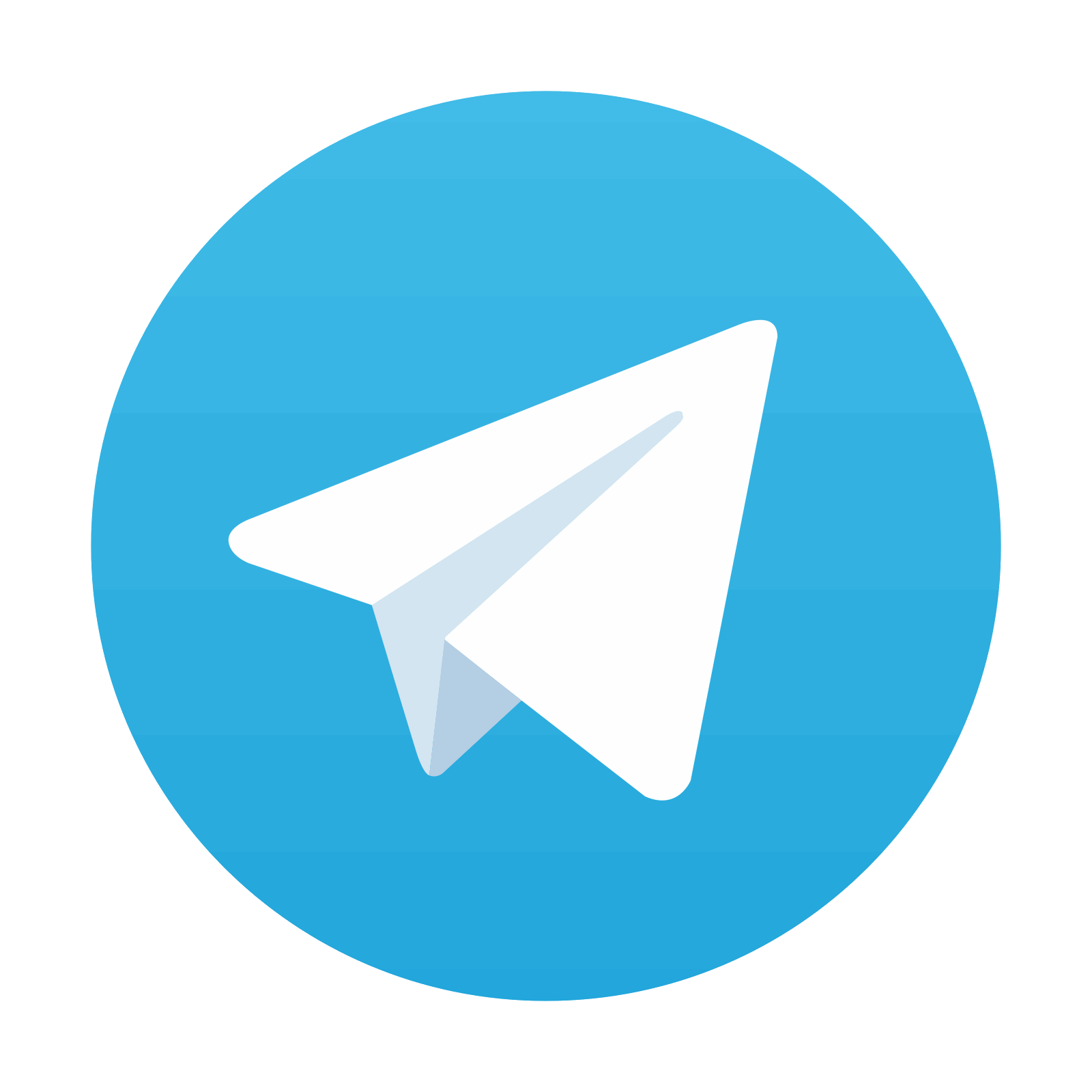
Stay updated, free articles. Join our Telegram channel

Full access? Get Clinical Tree
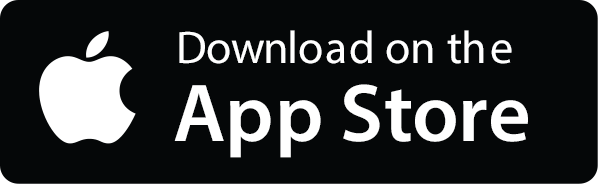
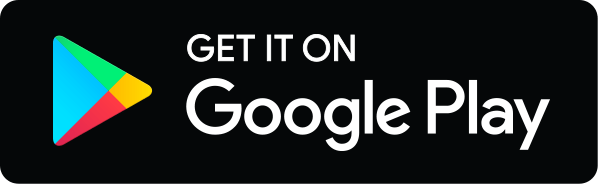