Summary of laser-tissue interactions in urology.
Holmium:YAG and FREDDY laser lithotripsy.
Laser treatment of benign prostatic hyperplasia using high-power Holmium:YAG and KTP (Greenlight) lasers.
Laser incision of urethral and ureteral strictures.
Other laser applications in urology for treatment of penile carcinoma, partial nephrectomy, tissue welding,…etc.
Introduction
Lasers have had a major impact in urology especially for the treatment of urinary stone disease and benign prostatic hyperplasia. |
Medical laser systems have had a reputation for being large, difficult to use, and expensive. However, recent technological advances have resulted in the commercial availability of clinical lasers that are more compact, powerful, affordable, and user-friendly. Clinical expertise in laser surgery has also improved, and as a result, the impact of lasers on the practice of urology has been significant.
Solid-state lasers, such as the potassium-titanyl-phosphate (KTP) or frequency-doubled neodymium yttrium-aluminum-garnet (Nd:YAG) laser, and holmium:YAG (Ho:YAG) lasers, have replaced older gas and dye lasers for soft tissue procedures and laser lithotripsy. Continued development of flexible endoscopes combined with the availability of small, flexible, biocompatible, inexpensive, and disposable optical fibers, have also created new opportunities for minimally invasive laser procedures in the urological tract.
Laser procedures in urology are based on a wide range of mechanisms for laser-tissue interactions, including photothermal, photomechanical, and photochemical (Table 1). Examples of photothermal applications include laser ablation of soft tissues for treatment of benign prostate hyperplasia (BPH), strictures, bladder cancer, laser tissue welding, and long-pulse laser lithotripsy. Photomechanical applications include short-pulse laser lithotripsy, and photochemical applications include photodynamic therapy (PDT) for treatment of bladder and prostate cancer.
Table 1
Mechanisms of laser-tissue interaction
Interaction | Mechanism | Lasers | Applications |
---|---|---|---|
Photothermal | Light → heat | Holmium:YAG, KTP | Benign prostatic hyperplasia, bladder tumors, strictures, long-pulse lithotripsy, soft tissue coagulation, tissue welding |
Neodymium:YAG | |||
Photomechanical | Light → shock wave | Dye, FREDDY | Short-pulse lithotripsy |
Photochemical | Light → chemical reaction | Dye, diode | Photodynamic therapy for bladder and prostate cancer |
This article reviews in detail the two most common clinical applications of lasers in urology – laser lithotripsy for fragmentation of urinary stones and laser ablation of the prostate for treatment of benign prostatic hyperplasia (BPH), and also covers other laser applications such as incision of strictures and tissue welding. The development of new experimental laser technologies for potential use in urology is also discussed.
Laser Lithotripsy
Introduction
Holmium:YAG laser is the most common lithotripter for fragmenting urinary stones located in the ureter, kidney, and bladder, and is a multiple-use laser in urology. FREDDY laser represents a compact, solid-state alternative to the older dye laser for short-pulse, photomechanical-based lithotripsy. |
The first laser to be used for the fragmentation of urinary calculi was the continuous wave ruby laser, the subject of in vitro experiments in the 1960s.1 The ruby laser destroyed kidney stones by a photothermal effect, as stones were simply heated until their melting point was reached. As a consequence, extreme heat was generated at the stone surface, making the ruby laser impractical for clinical use. Subsequent development of the pulsed dye laser marked the beginning of the clinical use of lasers to fragment urinary calculi.2 The application of pulsed energy to the stone surface yields a finite zone of energy density, with little heat dissipation into the surrounding fluid and tissue.
The first widely available pulsed dye laser was the coumarin laser, which relied on the excitation of green coumarin dye by light energy. The coumarin laser was a significant advancement in the field of laser lithotripsy, as it could fragment stones of all composition except for cystine, and the energy could be transmitted through small, 200 μm, quartz fibers. Although success rates of 80–95% were reported, the coumarin laser had a number of drawbacks, including the high initial cost of the device, disposal of the toxic coumarin dye, and cumbersome eye protection.
The Holmium:Yttrium-Aluminum-Garnet (Ho:YAG) laser was developed to replace the pulsed dye laser, and is now generally considered to be the standard laser for stone fragmentation (Table 2). The holmium laser is a solid-state device, and is smaller, less costly to operate, and requires less maintenance than the previous pulsed-dye lasers. The holmium laser has a long pulse duration of approximately 500 μs which promotes stone fragmentation by two mechanisms: photomechanical, via shock wave generation, as well as photothermal, via heat generation. The primary mechanism of stone fracture is the photothermal effect.3 The holmium laser wavelength is strongly absorbed by water, and its depth of penetration is relatively shallow; consequently, the laser can be discharged at a distance of even 0.5–1 mm from soft tissue with no resultant collateral damage.4
Table 2
Technical specifications for lasers currently used for lithotripsy
Laser parameters | Holmium:YAG | KTP/Nd:YAG |
---|---|---|
Wavelength (nm) | 2,120 | 532/1,064 |
Operation mode | Long-pulse | Short-pulse |
Pulse energy (J) | 0.4–3 | 0.160 |
Pulse length (μs) | 350–700 | 1.2 |
Repetition rate (Hz) | 5–20 | 1–20 |
Average power (W) | 30 | 3.2 |
Electrical specifications | 110 V, 15 A | 110 V, 6 A |
Weight (kg) | 84 | 45 |
The frequency doubled double-pulse Neodymium:YAG (FREDDY) laser is the result of efforts to reduce the size, cost, and maintenance of clinical laser systems (Table 2). The FREDDY laser incorporates a KTP crystal into the resonator of a Nd:YAG laser, so that when the device is activated two laser pulses are produced simultaneously. The stone fragmentation mechanism is photomechanical, as one laser pulse generates a plasma bubble and the second laser pulse excites to violently collapse and release a shockwave at the surface of the stone. Although initial work with the FREDDY laser was encouraging, more recent evidence suggests that certain types of calculi do not respond well to this technology.5 For this reason, the FREDDY laser has not achieved the widespread utilization of the holmium laser.
Indications and Contraindications
Indications Urinary stones less than 1.0–1.5 cm in diameter Contraindications Presence of untreated infection. |
In general, any stone within the urinary tract may be treated with the holmium laser. However, holmium laser lithotripsy as a treatment modality for renal calculi is sensitive to stone size, and for large stone burdens the procedure’s efficacy can be markedly reduced. For upper urinary tract calculi less than 1.0–1.5 cm, ureteroscopic holmium laser lithotripsy is a reasonable treatment option.6 However, when the stone burden increases beyond this size limit, the likelihood of achieving a stone-free outcome is reduced, and such patients may be best approached in a percutaneous fashion using a rigid lithotrite. For patients with bladder calculi, the holmium laser can be used to fragment stones of any size. In general the treatment of bladder stones is more efficient, as larger laser fibers can be used, which allow the transmission of greater amounts of energy.
The only true contraindication to holmium laser lithotripsy of urinary calculi is the presence of untreated infection, as life-threatening sepsis may result. Otherwise, there are no other specific contraindications to holmium laser lithotripsy. Indeed, even patients receiving anti-coagulation therapy have been reported to have successfully undergone holmium laser treatment of urinary calculi.7
Technique
Fiber Selection 550 or 1,000 μm fiber for use with rigid cystoscope in bladder. 365 μm fiber for use with rigid ureteroscope in ureter. 200 μm fiber for use with flexible ureteroscope in kidney. Energy Settings Pulse energies of 0.6–1.2 J. Pulse rates of 5–15 Hz. |
Prior to fragmenting a stone with the holmium laser, the treating physician must first select the appropriate fiber for delivery of the laser energy as well as the proper energy settings of the laser itself.8,9 Holmium laser fibers are typically available in 200, 365, 550, and 1,000 μm diameters, either in end- or side-firing configuration. Laser lithotripsy is most effective with an end-firing fiber rather than a side-firing fiber. The diameter of the fiber to be used typically depends on the location of the targeted stone and the endoscope used to access the stone. For patients with stones in the urinary bladder, a rigid cystoscopic instrument is used to visualize the stone, and either a 550 or 1,000 μm laser fiber is used to deliver the laser energy to the stone. The larger fiber size enables greater energy transmission to the stone, and irrigation flow through the cystoscopic instruments is not affected by these larger fibers. Ureteroscopes are much more miniaturized, and irrigation flow through these endoscopes is more sensitive to the size of the laser fiber. In general, a 365 μm fiber can be easily passed through a rigid ureteroscope with minimal effect on the flow of irrigant. However, a 365 μm fiber will attenuate the flow of irrigation through a flexible ureteroscope, as well as impair the deflection of the flexible endoscope. For these reasons, a 200 μm fiber should be used when a flexible ureteroscope is required to access the stone, and a 365 fiber should be used when a rigid ureteroscope is required to access the stone.
Lithotripsy with the holmium laser depends on both the pulse energy as well as the pulse rate. For the fragmentation of stones, pulse energies of 0.6–1.2 J and pulse rates of 5–15 Hz are necessary. To maximize the laser’s margin of safety, treatment usually begins with a pulse energy of 0.6 J and only increases as needed to fragment the stone. The laser fiber should be applied to the targeted stone in a paintbrush fashion, which vaporizes the stone rather than drilling into it. The endpoint of laser lithotripsy is generally either when stone fragments are small enough that they might be expected to pass spontaneously or until they can be safely extracted with a basket or grasping device.
Adverse Events
Injury to urothelial tissue and tissue perforation. |
In general, the Holmium laser is one of the safest intracorporeal lithotrites available for stone fragmentation. The most significant adverse event is injury of the urothelial tissue adjacent to the treated stone. The depth of tissue penetration of the Holmium laser is 0.5–1.0 mm, so in most cases such injuries may be managed conservatively, although a ureteral stricture may be a long term consequence of such an event. The Holmium laser does produce a weak shockwave, so in some cases stone fragments can be retropulsed and migrate away from the endoscope, which may increase the complexity of the procedure. Eye protection is required for operators of the Holmium laser, although at the energy levels used for the fragmentation of calculi the operator’s cornea would be damaged only if it was positioned at less than 10 cm from the laser fiber. A theoretical side effect of holmium laser lithotripsy is the production of cyanide when uric acid stones are treated, although this has never been encountered in clinical experience.
Future Directions
Development of smaller (150 μm) fibers that are more flexible and do not impede endoscope bending. More rapid and efficient fragmentation of larger urinary stones using novel solid-state and fiber lasers (e.g., Erbium:YAG and Thulium fiber lasers). |
The field of laser lithotripsy is advancing in two different directions: improvements to the existing Holmium laser platform, and development of novel laser platforms. The most significant improvement in Holmium laser lithotripsy will likely come from improved delivery fibers. At present, the smallest fiber in widespread use, the 200 μm fiber, impedes deflection of a flexible ureteroscope by up to 20°. As smaller laser fibers, such as 150 μm fibers, are produced, it is likely that this effect on endoscope deflection will be further reduced. The fracture of a laser fiber inside of an endoscope can result in a catastrophic failure of the scope, and when this occurs the fiber-optic bundles that transmit images and light are generally destroyed. Future efforts towards maximizing fiber durability may reduce these events.
The Erbium:YAG laser has recently been tested for fragmentation of urinary calculi.10 The erbium laser wavelength of 2,940 nm is more strongly absorbed by water than that of the holmium laser wavelength at 2,120 nm, which has translated to a 2–3 fold increase in efficiency for fragmenting urinary stones in an in vitro setting. However, there are several limitations of the erbium laser that prevent its immediate use in the clinic. The erbium laser wavelength cannot be transmitted through standard low-OH silica fibers, and mid-infrared fibers are needed which are typically less flexible, more expensive, and less biocompatible than silica fibers. The high-power thulium fiber laser has also been tested for lithotripsy, and, unlike the erbium laser, it may be used with standard silica fibers.11 The thulium fiber laser has several potential advantages over the solid-state holmium laser, including more efficient operation, more flexible operating parameters, and a smaller beam diameter for easier coupling into small optical fibers for use in flexible endoscopes.
Benign Prostatic Hyperplasia
Introduction
Holmium:YAG and Frequency-doubled Neodymium:YAG (KTP or “Greenlight”) are most commonly used lasers for treatment of BPH. Higher power Holmium:YAG (100 W) and KTP (120 W) lasers have recently become available for more rapid treatment of BPH. |
As the population ages, the prevalence of BPH, and its accompanying voiding symptoms, is increasing.12 The natural history of BPH is often one of progression, with mild symptoms becoming more bothersome over time. Indeed, among 50-year-old men, the lifetime incidence of surgical or medical intervention for BPH is estimated to be 35%. Transurethral resection of the prostate has historically been the most common surgical intervention for men with the symptoms of BPH. Over the past decade, several laser based technologies have been developed for the treatment of men suffering from BPH.
In general, the popularly utilized laser platforms for the treatment of BPH are the KTP laser and the Holmium:YAG laser (Table 3), both of which rely on an ablative mechanism of action in which prostate tissue is removed either through incision or vaporization by elevating the tissue temperature well above 100°C. The 532 nm wavelength of the KTP laser is more strongly absorbed by blood, resulting in a shallow optical penetration depth in the tissue (approximately 0.8 mm) (Fig. 1), and efficient tissue ablation with a thermal coagulation zone of approximately 1–2 mm. The KTP laser procedure, pioneered by Malek and associates,13 is termed Photoselective Vaporization of the Prostate (PVP) because the laser wavelength is not absorbed by water, but rather selectively absorbed by hemoglobin in the tissue, resulting in direct vaporization of the prostate. During PVP, a cavity is created after laser tissue vaporization, which is similar to TURP. With the recent commercial availability of higher power, 80 W KTP lasers, such as the “Greenlight” laser, higher tissue vaporization rates have been achieved in a virtually bloodless procedure. Operative times have decreased significantly compared to earlier studies with the lower power 40 W and 60 W KTP lasers. As a result, this procedure has recently become one of the preferred methods for laser treatment of BPH, and early studies have demonstrated results equivalent to TURP.14,15 The long-term effectiveness of the procedure has yet to be determined. The most recent version of the KTP laser provides even more power, 120 W, although long-term evaluations of its clinical performance have not yet been reported.
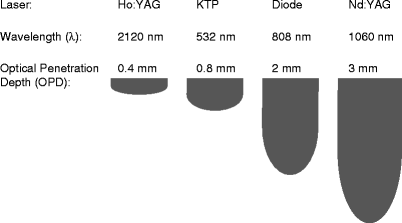
Table 3
Technical specifications for lasers used in treatment of BPH
Laser parameters | Holmium | KTP |
---|---|---|
Wavelength (nm) | 2,120 | 532 |
Operation mode | Pulsed | CW |
Pulse length (μs) | <600 | – |
Repetition rate (Hz) | 5–50 | – |
Average power (W) | 100 | 120 |
Electrical specifications | 220 V, 30 A | 220 V, 30 A |
Weight (kg) | 155 | 152 |
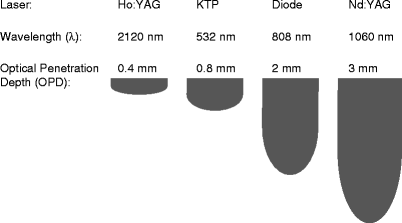
Fig. 1
Optical penetration depths in soft tissue as a function of wavelength for several common lasers used in urology
The application of the Holmium:YAG laser to BPH has evolved in stages. Initially, holmium laser vaporization of the prostate (HoLAP) was developed using low-power holmium lasers, but it was found to be a time-consuming procedure limited to use in smaller prostates. Holmium laser resection of the prostate (HoLRP) was then developed, but the technique was difficult to learn. During HoLRP, the holmium laser with a wavelength of 2,120 nm is used to provide precise tissue cutting with an optical penetration depth of only 0.4 mm, thus producing a thin coagulation zone. The prostatic lobes are resected and removed with a syringe or grasping loop. This procedure is also slow, requires long laser irradiation times, and is not practical for large prostates.
However, with the recent introduction of higher power holmium lasers with output powers up to 100 W, HoLAP has been re-explored, as procedure times could be reduced so that they are comparable to KTP laser ablation. As of yet, however, there are no published studies directly comparing the 80 W KTP and the 100 W Ho:YAG lasers for vaporization of prostate tissue, so it is unclear which technology provides an advantage for this type of procedure. Further complicating matters, both lasers operate based on different mechanisms of laser-tissue interaction. The KTP laser radiation can be transmitted easily through water in a noncontact mode, because the laser radiation is selectively absorbed by the hemoglobin in the tissue. On the contrary, the holmium laser radiation is highly absorbed by the water in the tissue, and although it can be used in noncontact mode due to the “Moses effect” (a cavitation bubble forms at the end of the fiber tip due to the short laser pulse and effectively “parts the waters”), the efficiency of tissue vaporization may be reduced.
The most recent evolution of the holmium laser procedure is termed Holmium laser enucleation of the prostate (HoLEP) and was pioneered by Gilling and colleagues.16
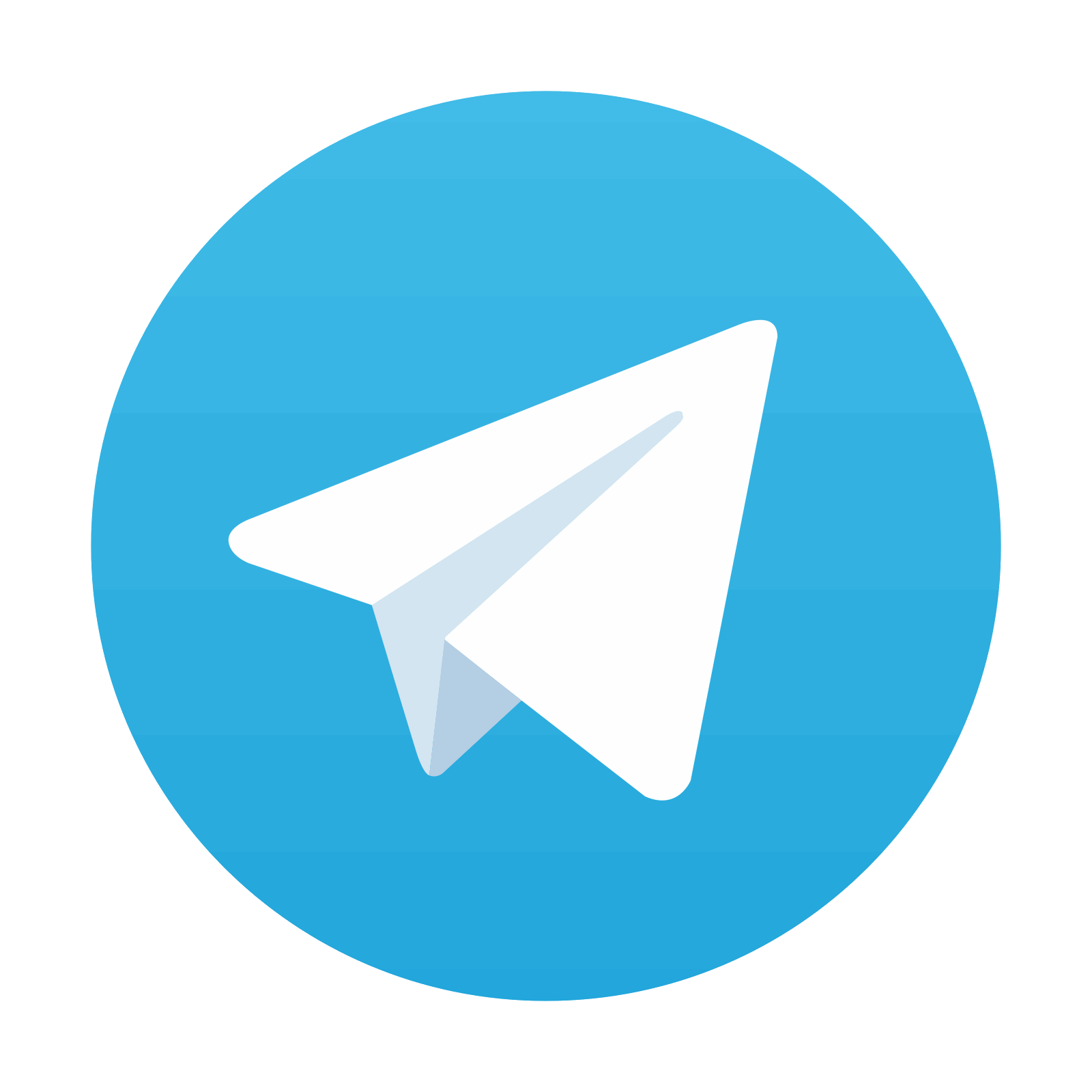
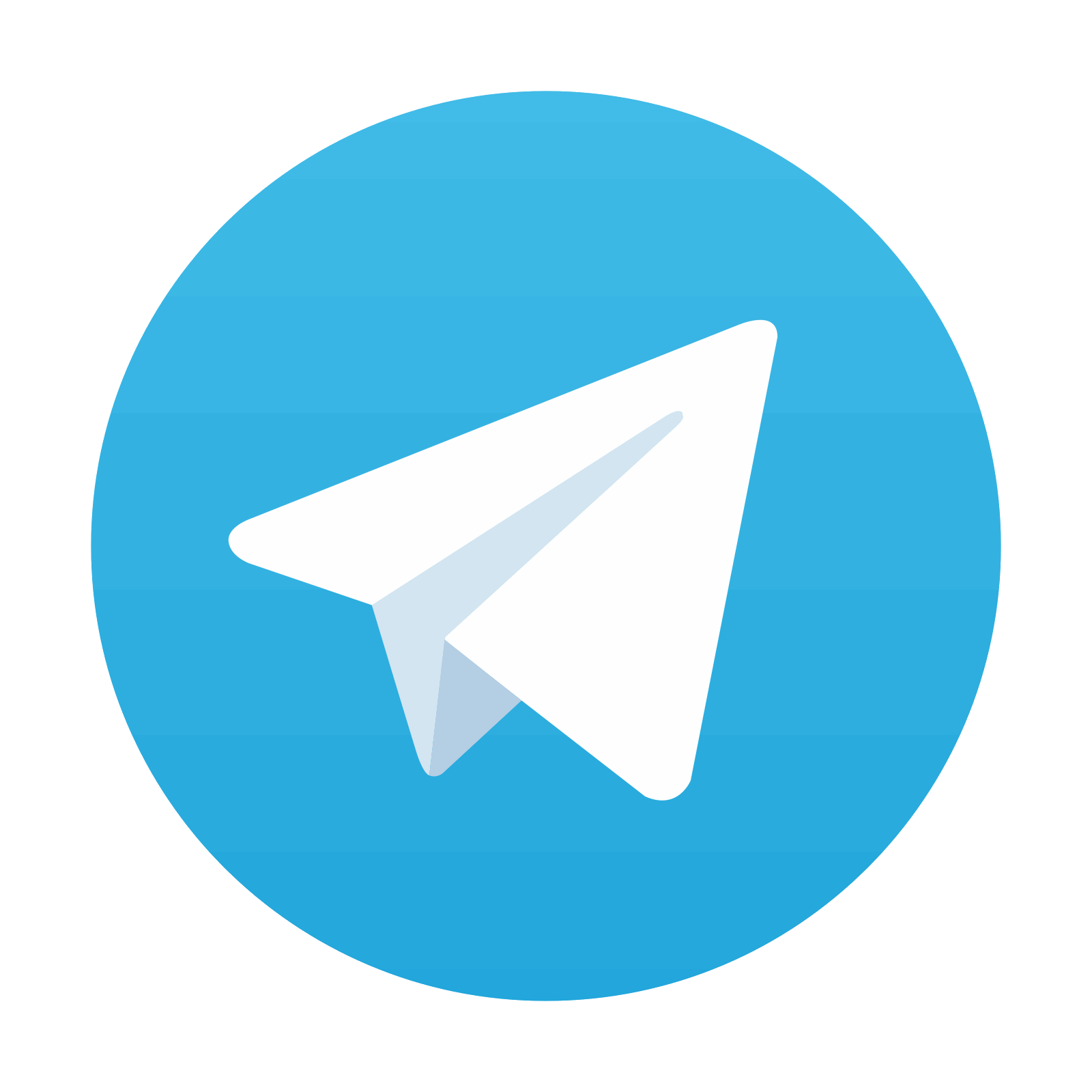
Stay updated, free articles. Join our Telegram channel

Full access? Get Clinical Tree
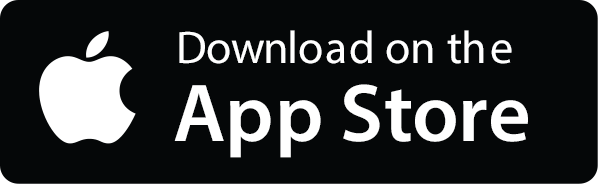
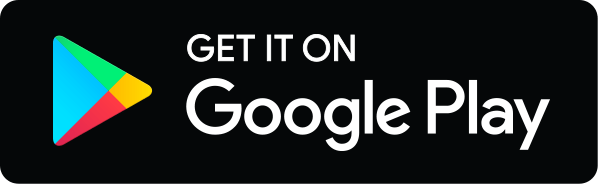