Refractive laser technology is used for correction of myopia, hyperopia and astigmatism.
Laser vision correction can be performed on the surface the cornea after removing the epithelium or deeper into stroma under a hinged corneal flap.
Proper candidate selection is essential to minimize the risk of complications.
Corneal collagen cross-linking with ultraviolet light and riboflavin (a photo sensitizer) is a relatively new treatment modality for a variety of corneal keratectatic disorders.
History
Laser technology is only around half a century old. The first experimental laser, demonstrated by Maiman in 1960, was produced by a ruby crystal powered by a flashlamp.1 However the first use of laser in the field of refractive surgery was demonstrated in 1983 by Trokel and Srinivasan who showed that argon-fluoride excimer laser of 193 nm could cleanly remove corneal stromal tissue with minimal damage to adjacent stroma.2 This technology was developed for ophthalmic use after being applied to etch computer chips for IBM in the late 1970s. Initially, the laser was used to create radial keratotomy incisions3,4; later it was used to ablate the corneal tissue in the central visual axis. This later procedure was termed photorefractive keratectomy (PRK) by Trokel and Marshall. The first PRK in a seeing human eye was performed by Mc Donald and coworkers in 1988.5 Since its inception, refractive laser technology has grown exponentially. In the US, The Food and Drug Administration (FDA) first approved the excimer laser for the treatment of mild to moderate myopia in October 1995. Later, it was approved for correction of hyperopia and astigmatism.
LASEK (laser-assisted subepithelial keratectomy) and epi-LASIK are modifications of the PRK procedure in which the corneal epithelium is preserved. The epithelium in such cases is displaced prior to the surface ablation, then replaced after laser application.
The term Laser (-assisted) in-situ keratomileusis (LASIK) was introduced by Ioannis Pallikaris and colleagues in 1988.6 LASIK involves the creation of a hinged corneal flap followed by ablation and reshaping of the underlying stromal tissue with an excimer laser beam. In most settings, the hinged corneal flap is created by an automated keratome. The keratome-laser combination was reported in 1990 separately by two investigators, viz. Burrato, who performed photokeratomileusis (PKM), and by Pallikaris, who performed LASIK.7 Burrato’s technique involved fashioning of a free keratomileusis cap and performing PKM on the posterior (stromal) aspect of the cap and replacing it. Pallikaris’ technique involved raising a hinged cap and treating the underlying stromal bed with an excimer laser.7 While the results of PKM were not as promising as LASIK, the LASIK hinged corneal flaps were safer and resulted in more accurate flap realignment.
Surface Ablation – PRK (Photorefractive Keratectomy) /LASEK (Laser Subepithelial Keratomileusis)/Epi-LASIK
Surface ablation has traditionally referred to PRK and requires removal of the corneal epithelium. This can be performed mechanically (scraping), chemically with alcohol (15–20%), or with the excimer laser (transepithelial ablation). However, it is most commonly performed by using dilute alcohol (commonly 15–20% ethanol is used) to loosen up epithelial cells, removing the epithelium (sometimes as a sheet), followed by laser ablation of the subepithelial stroma. After the laser procedure is completed, the epithelial sheet is either replaced (LASEK) or discarded (PRK). The theoretical advantages of LASEK over PRK include decreased post-operative discomfort, reduced risk of scarring and faster visual recovery. A study by Wissing et al. demonstrated reduced keratocyte loss with LASEK when compared to PRK in an animal study.8 However, more clinical data is needed to conclusively demonstrate that LASEK has significant advantages over PRK. On the other hand PRK may be more advantageous than LASEK in situations like anterior basement membrane (Cogan’s) dystrophy,9 epithelial erosions or epithelial scaring, where it may be preferable to discard the diseased epithelium. In most cases, the choice of one procedure versus the other is largely dependant on surgeon preferences.
The principal advantage of surface ablation is the avoidance of flap-related LASIK complications, for e.g., flap dislocation, flap folds, button-hole formation, etc (discussed in details in section “Complications of Laser Refractive Surgery”). Moreover, surface ablation may be the preferred approach in certain situations. These include certain occupations in which the risk of flap trauma is persistently elevated, for e.g., in contact sports like basketball and rugby, military personnel, etc. Patients with a history of systemic herpetic infections (a relative contraindication) may be better suited for surface ablation, which theoretically severs less number of corneal nerves as compared to LASIK and may therefore have lesser propensity to reactivate the virus. For similar reasons, it is generally speculated that surface ablation has less of a tendency to induce dry eyes when compared to LASIK. Moreover, in patients with a prior history of flap abnormalities with attempted LASIK, it may be safer to perform surface ablation rather than run the risks of recutting the flap such as flap maceration and flap loss.10 Several factors, such as lack of reproducibility of corneal flap thickness even when the same microkeratome is used11 and variations in intensity and duration of microkeratome suction,12 predispose to serious flap complications during recutting the flap.
The major advantages of LASIK over surface ablation are earlier post-operative visual recovery, less post-operative discomfort, less risk of haze esp. when associated with higher level of refractive error correction and shorter duration of post-operative steroid treatment.9 Moreover, LASIK may be associated with less regression when compared to surface ablation for higher levels of correction.9
A variation of LASEK, termed epi-LASIK was applied by Pallikaris et al. who used an automated separator to remove the corneal epithelium mechanically, without the use of alcohol.13 They suggested that this technique would provide increased post-operative comfort and decreased haze formation as compared to PRK. Histological studies seem to show better preservation of the epithelial sheet when compared to LASEK.14
LASIK
Laser in-situ keratomileusis (LASIK) is a two-step procedure that involves creation of a hinged corneal flap followed by ablation and reshaping of the underlying stromal tissue with an excimer laser (‘excited dimer’) beam. This is an argon fluoride (AFl) laser that operates at 193 nm in the ultraviolet spectrum and was first used for etching computer chips.2 This laser wavelength allows ophthalmologists to surgically reshape the cornea through a series of mirrors and lenses in an attempt to circumvent the need for corrective lenses. There are three general types of delivery systems for the excimer lasers, depending on the size of the beam : broad beam, scanning slit, and small beam. The broad beam laser is the one more commonly used for corneal reshaping through an iris diaphragm. Due to the high energy per photon of the excimer laser beam, the ablation of the carbon-carbon bonds at the corneal surface allows for an accurate tissue removal of each layer with minimal penetration and therefore damage to adjacent tissues.
The first step in LASIK is creation of a hinged corneal disc (i.e., the ‘flap’). This can be created by an automated microkeratome or more recently and with increased precision with the Intralase® femtosecond laser (Intralase Corp., Irvine, CA), an infrared YLF:glass (Nd:glass) laser which operates at 1053 nm15 (Fig. 1).
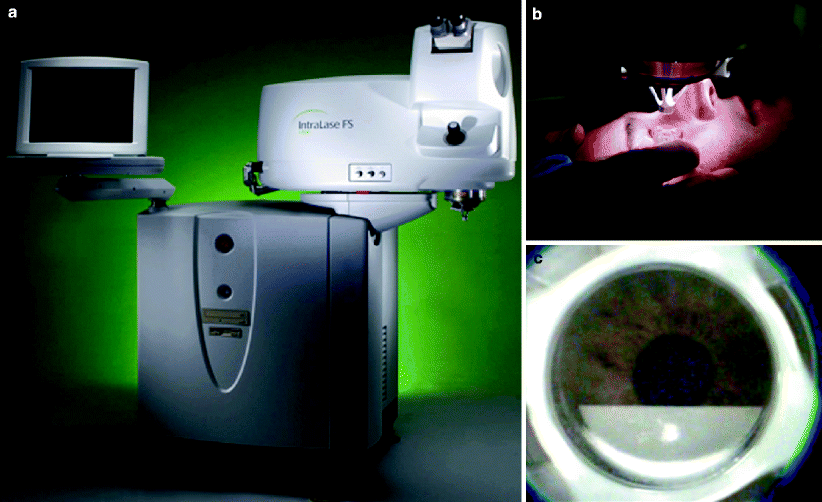
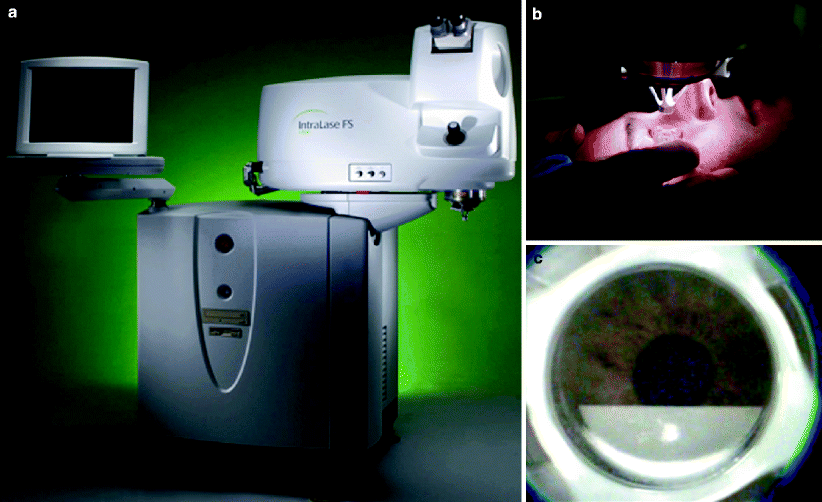
Fig. 1
(a) IntralaseTM Femtosecond laser unit. (b) Intralase suction ring placement with docking of the applanation cone. (c) LASIK flap creation with IntralaseTM using a raster scan pattern
Microkeratomes have greatly evolved in terms of ease of use, reliability and safety profile since the earlier original versions. Jose Ignacio Barraquer in 1958 unveiled his first manual microkeratome, which was designed for keratophakia and freeze keratomileusis.16 Over time, this technique was evolved for obtaining a smoother technique to cut through the corneal stroma. Microkeratomes come in manual, semiautomated, and automated models. Some are even disposable. Typical components of a standard microkeratome include the motor for translational movement, head of the microkeratome bearing the blade, applanator lens to measure the diameter of the exposed cornea, vacuum fixation ring to secure the eye, flat stop ring to limit the movement of the microkeratome head through the fixation ring and a foot switch to operate the microkeratome movement. Although much improvement has been made since the earlier days in mirokeratome design, modern day microkeratomes are not devoid of complications. Most of the flap related complications during LASIK (discussed in section “Complications of Laser Refractive Surgery”) are related to the use of microkeratomes. These complications depend on many factors, including surgeon experience and microkeratome safety features. Although there is a steep learning curve, complications decrease over time with increasing surgeon experience. Most modern microkeratomes have inbuilt safety features such as alarms, automatic shut down buttons and suction indicators.
With the advent of the femtosecond laser, creation of the LASIK flap has become much safer and simpler (Fig. 1). The flap can now be created optically with the near infrared laser, focused at a set depth within the corneal stroma. The laser fires at a rapid rate with pulse duration in the range of femtoseconds. This results in vaporization of corneal tissue by the process of photodisruption, leading to formation of plasma and shockwave. The expansion of plasma results in a resection plane which ultimately leads to creation of a lamellar flap. This flap still requires dissection of the lamellar plane prior to lifting the flap for treatment with the excimer laser. Thus, more uniform flaps with consistent thickness can be created. Also this laser system allows the refractive surgeon to fashion thinner flaps that have the advantage of preserving greater amounts of residual stromal bed. LASIK flap creation with femtosecond laser is growing in the U.S. and principally hindered by the cost of the laser.
Before the corneal lamellar flap is created, gentian violet surgical landmarks spanning the hinged flap and the peripheral cornea are placed and the flap is folded over to expose the stromal bed. The excimer laser (Fig. 2) is then used to reshape the corneal stroma to achieve the desired correction of refractive error. Both the size of the optical zone as well as the depth and profile of the laser ablation are important determinants of the correction achieved. Small or decentered ablation zones can result in problems of glare and haloes at night when the pupils may dilate beyond the functional optical zone. Deeper ablations can distort the corneal surface and can predispose to corneal flap striae or ‘mudcracks.’ In order to ensure adequate stromal hydration during LASIK, the laser treatment should be performed in a timely manner. Dehydration of the stroma can lead to overcorrection while over-hydration with BSS can dampen the effect of the laser. Most modern excimer lasers have a tracking mechanism (usually infrared cameras) based on the position of the pupil or the iris pattern in order to nullify the effect of torsion or slight movements of the eye during the time of laser treatment.
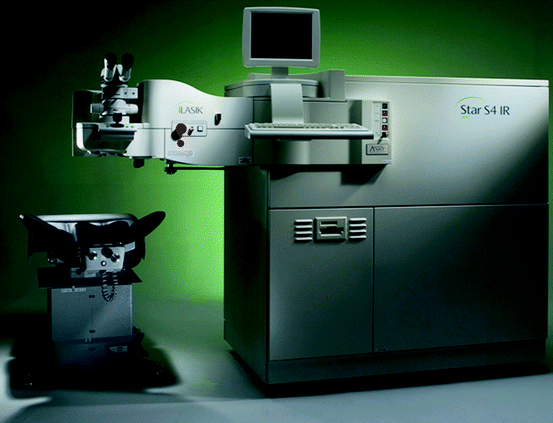
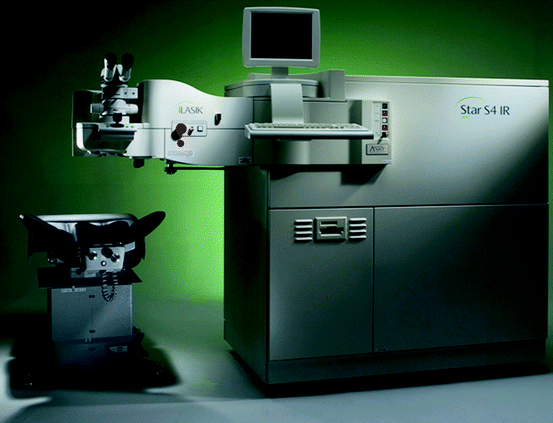
Fig. 2
Visx Star S4TM Excimer laser unit for laser vision correction
Once the ablation is complete, the undersurface of the flap along with the stromal bed is irrigated with balanced salt solution to remove any debris, and the flap repositioned onto the stromal bed. The interface is then allowed to dry for a few minutes. Proper adhesion of the flap is then confirmed. It is equally important to confirm adequate alignment of the flap with respect to the peripheral cornea using the pre-placed surgical landmarks as a guideline along with the configuration of the gutter between the flap and the peripheral cornea. At the end of the procedure, the patient should be re-examined under a slit lamp before being discharged and again the following day. One of the important reasons for such a close monitoring is that flap related complications such as flap folds or dislocation are easier to fix if detected early rather than later during the post-operative course. Post-operative regimen typically consists of topical antibiotics and topical steroids for a short duration along with non-preserved artificial tears as needed.
Custom Wavefront LASIK
Standard laser vision correction treats lower order aberrations only such as myopia, hyperopia, and astigmatism. However, higher order aberrations exist, such as coma, trefoil and spherical aberrations, that are not treated or even worsened by conventional excimer laser treatment (Fig. 3b). Induction or lack of treatment of these factors limit patient satisfaction after LASIK and include reduced contrast sensitivity that is largely accounted for by an increase in optical aberrations. Along with this, many patients experience night vision problems such as glare, haloes and starbursts postoperatively. Wave-front guided (Fig. 3a, b) LASIK is a relatively new development in the field of refractive surgery that aims to achieve better visual outcomes by correcting existing ocular aberrations.17
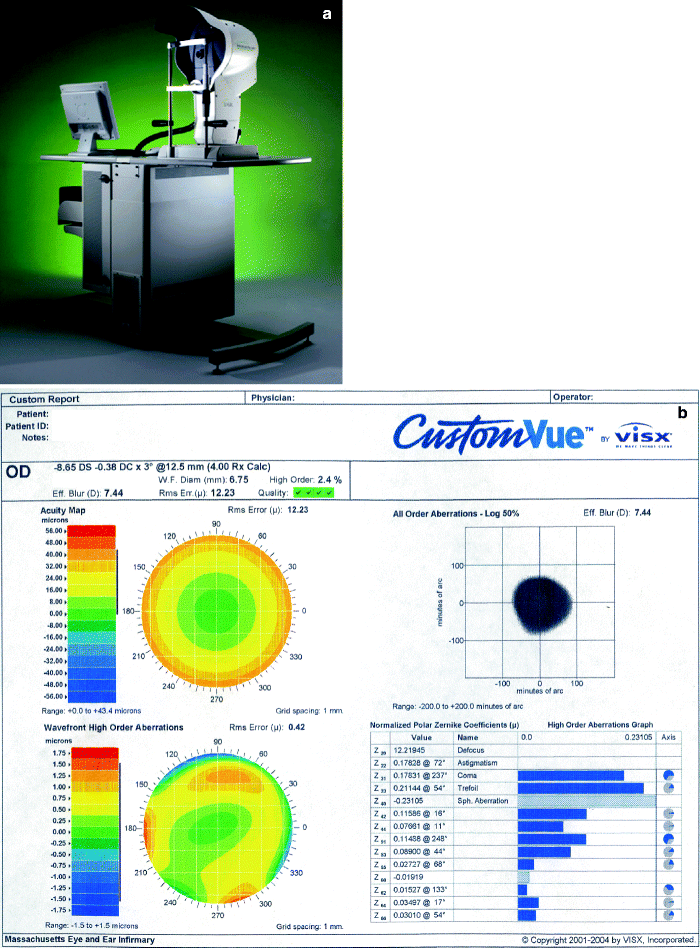
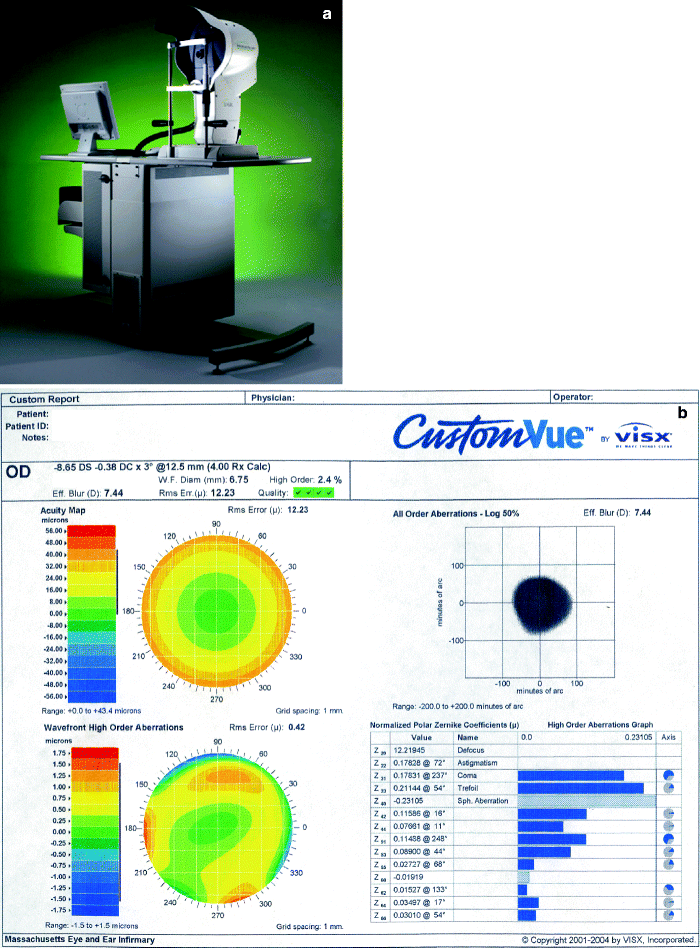
Fig. 3
(a) WaveScan® Wavefront Unit for optical aberrometry using a Hartmann–Shack technique. (b) Sheet generated by WaveScan® Wavefront Unit showing total and higher order aberrations
Wave-front guided LASIK aims to treat total ocular aberrations. This is in contrast to topography-guided ablation, which can treat ocular aberrations which arise from the corneal surface only and the conventional LASIK which cannot treat higher order aberrations. The wave-front guided ablation technology uses sophisticated computer software to display both low and higher aberrations to the surgeon. By canceling the phase differences within the wavefront, wavefront customized ablation aims to equalize the optical path of all rays entering the pupil from the object of focus to the fovea. Various factors, such as refractive index of the cornea, corneal curvature and optical zone blending are taken into account to optimize the ablation profile.18 In simple terms, conventional LASIK aims to treat a patient’s refractive error without taking into account other optical aberrations; therefore any two individuals with same eye-glasses prescription would have similar pattern of laser ablation, provided the other treatment parameters (for e.g., the size of the optical zone) are the same. Wave-front guided LASIK, on the other hand is much more customized to an individual’s hill of vision. It takes into account not just the patient’s eyeglasses’ prescription but also other optical aberrations which influence the quality of vision; therefore the pattern of ablation would be different amongst any two individuals with the same eye-glasses’ prescription.
Several studies show better contrast sensitivity and less night vision problems after wave-front guided LASIK.19 Some other studies, however, seem to suggest that wave-front guided LASIK cannot remove high-order aberrations but can reduce the increase in high-order aberrations after laser treatment when compared to conventional ablation.20 Despite its many advantages, every patient may not be a suitable candidate for wave-front guided laser treatment. This is because correction of large amounts of higher-order aberrations necessitates ablation of increased amounts of corneal tissues. Careful consideration of the benefits of customized ablation versus the risks of keratectasia due to inadequate residual corneal stromal bed thickness should be made, especially in patients with higher levels of refractive error and border-line thin corneas.21
Complications of Laser Refractive Surgery
Laser vision correction is not free of complications. Proper candidate selection is one of the most critical steps to minimize the risk of such complications. Nonetheless, despite adequate candidate selection, complications still occur in the best of the hands, and patients should be warned about the potential risks of this elective procedure. In addition to explaining the general risks, individual patients should be warned about the specific risks of particular complications based on their prescription and the configuration of the cornea and the pupils. Any co-existing pathology such as glaucoma, lens opacities, corneal dystrophies, dry eyes and diabetes should be clearly documented and explained to the patient. Table 1 lists the important pre-operative tests that are considered to be essential in order to adequately determine candidacy for refractive surgery in addition to a thorough history and meticulous clinical examination of the eye.
Table 1
Patient evaluation prior to refractive surgery
Contact lens users should discontinue the use of contact lenses for at least 10–14 days prior to evaluation. Hard and toric contact lens wearer longer. |
The following tests should be done on a day other than the day of surgery. |
Full ocular examination including fundus examination and motility assessment |
Refraction: both dry manifest and cycloplegic |
Keratometry |
Corneal topography |
Pupillometry |
Pachymetry |
Dry eye testing |
Wavefront aberrometry |
A full discussion of the associated complications and their management options is beyond the scope of this book. Herein, we discuss some the key complications which can be broadly divided into the following categories:
1.
Anatomic Complications
2.
Refractive Complications
