Applications of light in neurology and neurosurgery can be diagnostic or therapeutic.
Neurophotonics is the science of photon interaction with neural tissue.
Photodynamic therapy (PDT) has been attempted to destroy infiltrative tumor cells in tissue.
Spatially modulated imaging (MI) is a newly described non-contact optical technique in the spatial domain. With this technique, both quantitative mapping of tissue optical properties within a single measurement and cross sectional optical tomography can be achieved rapidly.
The ability to control the activity of a defined class of neurons has the potential to advance clinical neuromodulation.
Introduction
Applications of light in neurology and neurosurgery can be diagnostic or therapeutic. While the therapeutic effects of light are dependent mainly on the ablative power of photon energy in lasers, the diagnostic use of light has mainly been in the near infrared (NIR) domain. This is because NIR light has better tissue penetration than visible light, as well as its ability to obtain physiologic information based on differential absorption and scattering of NIR light during different physiological states. In this chapter we review the diagnostic and therapeutic applications of light in neurology and neurosurgery. In the last section we give a brief overview of novel and promising optical technologies of neurologic interest in development.
Neurophotonics
Neurophotonics is the science of photon interaction with neural tissue. Four major types of photon-neural interactions characterize neurophotonics1: (a) absorption (energy of the photon is transferred to the tissue, may be re-emitted or transformed in the form of heat), (b) phosphorescence (energy of the photon is released by the tissue with a delay in the form of a photon with similar or lower energy), (c) fluorescence (energy of the photon is released in part in the form of a lower energy photon, the rest being dissipated as heat or as another lower energy photon), and (d) scattering (the photon remains of the same energy and is merely deviated in its trajectory). Some photons proceed less disturbed in their trajectory and transmission of light through tissue is observed.
All these types of interactions occur to some degree in the brain and in other living tissues; however, for NIR light, the dominant events are scattering and absorption (Fig. 1). For both scattering- and absorption-type interactions, the frequency of occurrence may change as a function of neuronal activity.
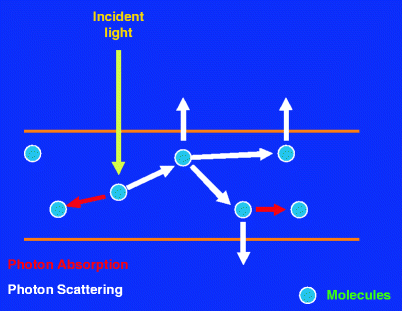
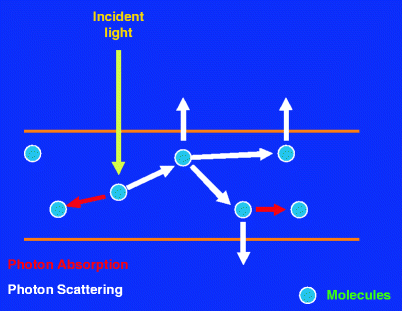
Fig. 1
Schematic showing the basic interaction of photons with tissue. Photon flux incident on tissue, when interacting with molecules can get partially absorbed leading to decreased flux (shown as thinning of arrows). The photons can also get multiply scattered before leaving the tissue giving rise to diffused light, which is a function of photon absorption and scattering and therefore depends on distance from the incident light. Transmitted light contains ballistic (not scattered) as well as snake photons (weakly scattered)
Changes in light scattering in relationship to action potentials were described by Cohen in 1972 and later by Stepnoski et al. in 1991.2,3 They are typically attributed to changes in the neuronal membrane reflectance due to the reorientations of molecules in the membrane and/or to volumetric changes that are determined by the movement of ions and water across and around the membrane. Changes in scattering and absorption properties are also likely to occur in the dendrites and soma during postsynaptic potentials. At the macroscopic level, the postsynaptic potentials, reflecting the activity of a much more extended portion of the neurons and integrating the activity over longer periods of time, are likely to dominate the measures. Several studies indicate that neural tissue becomes more transparent (i.e., less scattering) when it is activated and probably less transparent (i.e., more scattering) when it is inhibited, in a manner that appears very similar in time course to the local field potential recorded from the same area.4–6
Unlike scattering, changes in light absorption are, for the most part, directly related to the changes in the blood oxygenation levels and flow that occur with regional neural activation. Both oxy- and deoxy-hemoglobin have very characteristic and distinct absorption spectra that correspond to the different colors of arterial and venous blood (Fig. 2). This makes it possible to use a spectroscopic approach to measure changes in the concentration of oxy- and deoxy-hemoglobin as a function of neural activity.7,8
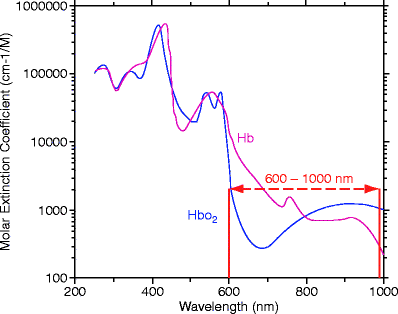
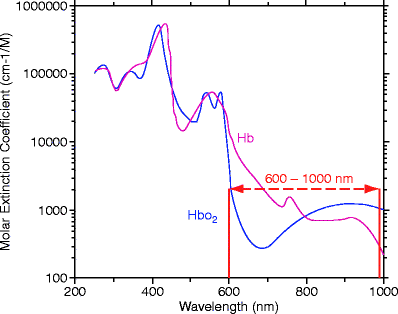
Fig. 2
Graph showing the molar extinction coefficients of oxy (HbO 2 ) and deoxy (Hb) hemoglobin. Between wavelengths of 600–1,000 nm the extinction coefficients of the two states of hemoglobin show low absorption with distinct spectral features. This provides a spectral window of good tissue penetrance of photons as well as allows better resolution of their individual chromophore concentrations from the Beer-Lambert Law
An example is a small increase in deoxy-hemoglobin concentration occurring within 1–1.5 s from the onset of neural activity (reflecting the increased oxygen consumption of active neural tissue), which is soon followed by a decrement in deoxy-hemoglobin concentration and a much larger increase in the oxy-hemoglobin concentration. This leads to the Blood Oxygen Level Dependent (or BOLD) functional MRI (fMRI) signal dependent on vasodilation.8
The relative changes in absorption and scattering during neural activity result from different physiologic phenomena.9 The hemodynamic-related absorption changes are much easier to observe than the neuronal phenomena. However, the scattering changes are a direct indication of neural activity, and therefore possess better temporal and spatial resolution, and more direct functional significance, than the hemodynamic phenomena. Different types of measurement methods may bias the recording system more toward one or the other of the two signals, which have been exploited in the development of novel optical modalities and imaging techniques described below.
Neurodiagnostic Applications of LASER and Light
NIRS and Photon Migration
Since Jobsis described the in vivo application of near-infrared spectroscopy (NIRS) to detect changes in cerebral hemoglobin oxygenation, several types of NIRS instruments have been developed.10 NIRS is widely used for the noninvasive monitoring of changes in chromophores such as oxy- and deoxy hemoglobin, lipid, and water from changes in the reflected light intensity. In the NIR region, the absorption spectra of these primary chromophores are different (Fig. 2). With determination of tissue absorption coefficients at multiple wavelengths (600–1,000 nm) and using appropriate modeling, information about absolute chromophore concentrations can be obtained (diffuse optical spectroscopy or DOS).
In this wavelength band, NIR light can penetrate the adult skull and propagate within the brain.1 Photons that propagate in the brain are strongly scattered elastically as they traverse several millimeters of tissue. Elastic scattering is characterized by the fact that the photon energy does not change. Since photons undergo multiple scattering events before they reach the detector, their trajectories are not straight lines; rather they resemble random walks. Multiple scattering of light which occurs as a consequence of spatial variation in refractive index influenced by cellular and extracellular optical densities, complicates quantitative measurements of light absorption in the NIR range. This complexity is a consequence of the uncertainty in optical path traveled by any given photon. Hence, a simple Beer’s law technique for deducing chromophore concentration cannot be simply applied. However, by using time-resolved or frequency-domain instruments, which effectively can be used, with appropriate selection of a model of light propagation, to deduce the average path length of detected photons, the effects of scattering can be separated from absorption. Thus absolute concentrations of tissue chromophores can subsequently be estimated in a given region of interest by application of the Beer-Lambert Law11 to the absorption coefficient. These techniques can provide quantitative measurements of optical and functional contrast between healthy and diseased tissue.20 NIRS offers an inexpensive and safe bedside monitoring tool.
Photon migration allows gathering information from relatively deep structures in highly scattering media. The light emitted from a source(s) diffuses in a random fashion through the scattering medium. However, because of statistical properties, the photons picked up by the detectors are more likely to have followed certain paths than others through the medium. If enough photons are used, the entire process of migration of photons from the sources to the detectors can be described accurately using mathematical formulations related to the diffusion process. This makes it possible to go beyond images of the first interaction of photons with the medium and produce images of structures that are up to a few centimeters deep into the medium.12
Frequency Domain Photon Migration
The frequency domain NIR system uses diode lasers as a light source with intensities modulated in the kHz to MHz range. In a typical embodiment, the brain is illuminated by one or more optical fibers. The reflected (diffusive) light is received by a detecting optical fiber, located at a small (several mm), well defined distance from the incident light source point and coupled to a silicon photodiode (Fig. 3). By solving the inverse problem for the diffusion equation for the light that travels through the tissue from source to detector, the optical properties and hence chromophore concentrations of the intervening tissue can be deduced.11 Alternative methods for recovering similar information have been developed and applied that rely on steady-state, non-modulated sources and multiple source-detector pairs, each pair separated by a well defined distance.13
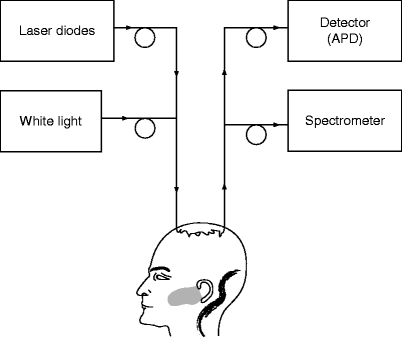
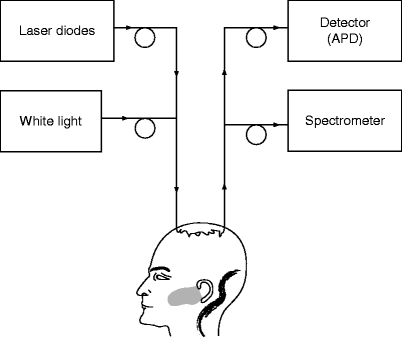
Fig. 3
Example of a broadband diffuse optical spectroscopy (b-DOS) system used to determine the optical properties (i.e. absorption and scattering) of brain tissue. This system combines Frequency domain (FD) photon migration (laser diodes and APD) and steady-state (white light and spectrometer) techniques. The system employs six laser diodes in the NIR range and avalanche photo diode (APD) detector. The APD detects the intensity-modulated diffuse reflectance signal at modulation frequencies after propagation through the brain. The optical properties are measured directly at each of the six laser diode wavelengths by using the frequency-dependent phase and amplitude data
Time Domain Photon Migration
In the time domain, sometimes know as time-resolved spectroscopy (TRS), source and detector are used and chromophore changes determined by calculating the emerging photon time of flight distribution.14 In this domain, ultrashort (picosecond) pulsed laser light is incident on the tissue. The detection process requires high speed, high sensitivity detector, which may be a time-correlated single-photon counting (TCSPC) system or a streak camera. Absorption results in a reduction of transmitted amplitude and pulse-width whereas scattering predominantly results in pulse broadening. These effects can be accounted for using an appropriate model of light propagation such as the diffusion equation, which allows the tissue optical properties to be separated and quantified.
Recently, spatial domain photon migration (spatially modulated imaging) has been introduced that enables quantitative wide-field spectral imaging of tissue structure and function.15 This technology is new and promising and may be particularly useful as an adjunct intraoperative imaging tool for cerebrovascular and neuro-oncologic surgery. A schematic diagram of spatial frequency domain imaging (SFDI) is shown in Fig. 4. The SFDI system is a relatively new concept that requires further validation. In principle, this method provides similar information to that provided by traditional DOS techniques, however it is able to do this in an imaging sense, using relatively inexpensive consumer grade electronics. Further description of the spatial frequency domain imaging is presented in section“Spatial Domain Imaging”.
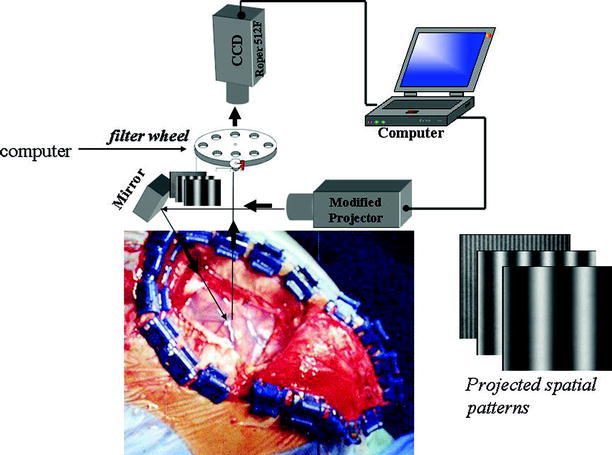
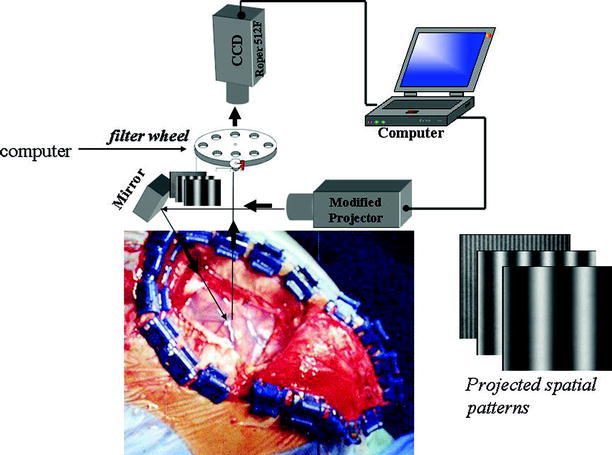
Fig. 4
Schematic diagram showing the intraoperative use of spatially modulated imaging. Periodic illumination patterns of various spatial frequencies with a 120°phase shifting between three adjacent patterns are projected onto the brain from a conventional projector controlled by a computer. The diffusely reflected pattern (the deformed fringe pattern) passes through near infrared (NIR) bandpass filters and is captured by CCD camera interfaced to a PC computer. The diffusion solution is then used as a model function in an inversion algorithm for determining separately the optical properties namely, absorption and reduced scattering coefficients over a wide spectrum range. Quantitative chromophore maps are then generated providing real time feedback to the surgeon
Several studies have been published on clinical applications of NIRS. Among other findings, these studies have described decrease in activation of the prefrontal cortex in traumatic brain injury patients,16 detection of cerebral ischemia during cerebrovascular and endovascular surgery,17–19 and measurement of cerebral hemodynamic response in infants.126
Intrinsic Signal Optical Imaging
Intrinsic Signal Optical Imaging (ISOI) is widely used for studying the hemodynamic response of the brain to stimulation. It is a functional neuroimaging technique that can map a large region of the cortex with high spatiotemporal resolution. The method uses a simple configuration containing a light source such as a light-emitting diode (LED) and a CCD camera to image the optical reflectance at this wavelength. The LED light in ISOI systems uses wavelengths (for instance 630 nm) that are maximally absorbed by the deoxygenated state of hemoglobin (Fig. 5). Hence, a decrease in reflected light indicates an increased absorption by deoxyhemoglobin. Therefore, ISOI provides relative changes in the local concentration of deoxyhemoglobin as a surrogate marker for neuronal activity.
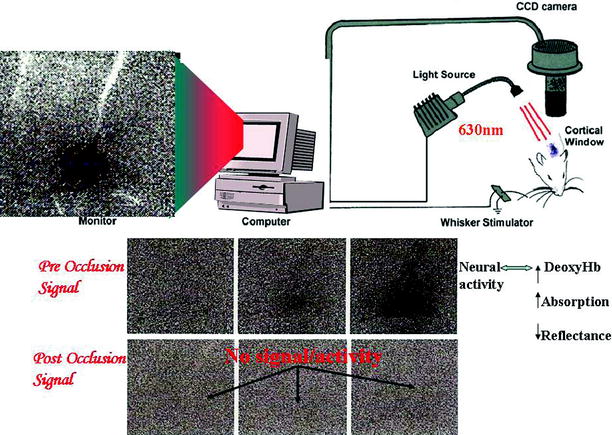
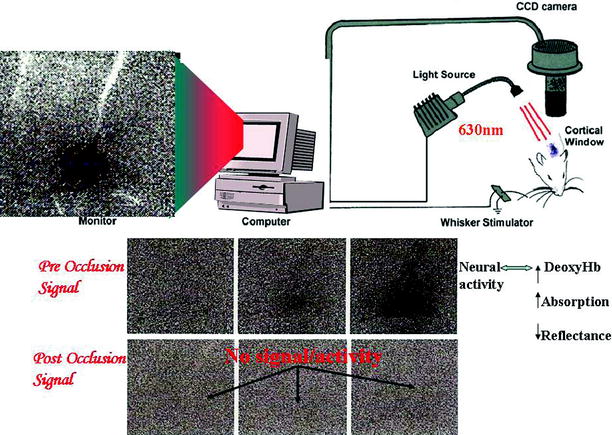
Fig. 5
Schematic diagram of an intrinsic optical imaging system (IOSI). Light is projected from a LED at wavelengths sensitive to the absorption of deoxyhemoglobin (e.g., 630 nm). Reflected light is captured on a CCD camera. Since, the amount of reflected light is inversely related to the regional deoxy hemoglobin concentration, functional activity can be mapped in the cortex. The tripanel grayscale figures show the results of IOSI in the rat barrel cortex during the first 1.5 s following contralateral whisker stimulation. Functional cortical activation (preocclusion) seen as the initial dip (darkening of the upper panel on the third image from left to right) is lost following MCA occlusion
Recently, a triphasic component has been described with a second increase in local deoxyhemoglobin concentration following the BOLD signal.21 The triphasic component constitutes an initial dip, overshoot and undershoot in light reflectance. The initial dip is from increased local deoxyhemoglobin secondary to neural oxygen metabolism from activation. In contrast, the overshoot is dominated by increase in oxyhemoglobin from neurovascular coupling. This signal is analogous to the BOLD signal observed and measured with fMRI. The undershoot signal represents the effort of the brain to come back to its normal state. IOSI studies in epilepsy have shown the presence of an epileptic dip analogous to the initial dip seen with functional activation.22 While ISOI’s main role has been in functional brain imaging in animals, it has potential in intraoperative functional mapping during neurosurgical procedures.23
Optical Coherence Tomography
Optical Coherence Tomography (OCT) is a novel imaging system analogous to ultrasound except that imaging is performed with light instead of acoustic waves. OCT measures light reflected from turbid structures. OCT is based on principles of low-coherence interferometry which allows detection of back scattered near-infrared photons from selected depths and rejection of photons being scattered from other layers. It is structurally based on the Michelson’s interferometer1 (Fig. 6). As for the reflection method, it is based on the first interaction (back-scattering) of photons in tissue. Unlike the reflection measures, however, it directly visualizes the depth at which this first interaction occurs.
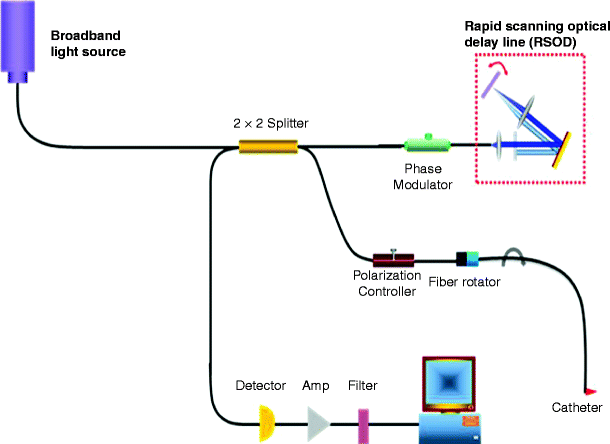
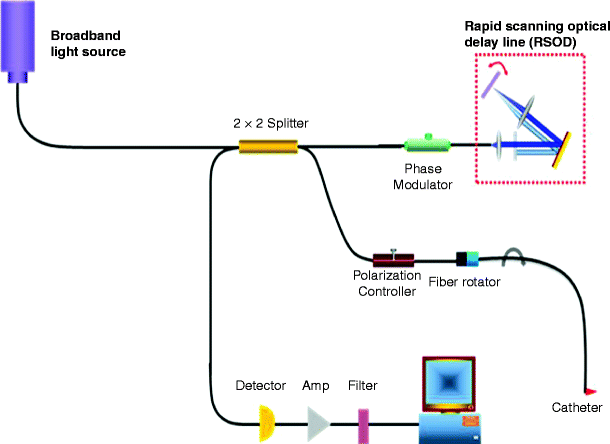
Fig. 6
Schematic diagram of a high speed, high resolution spectral domain OCT system which reconstructs vessel structure image. The system is constructed along the lines of a Michelson interferometer. Eighty percent of the incident power is coupled into sample arm while 20% is fed into a reference arm by a 1 × 2 fiber coupler. The reference power is attenuated by an adjustable neutral density attenuator for maximum sensitivity. Two circulators are used in both reference and sample arms to redirect the back-reflected light to the second 2 × 2 fiber coupler (50/50 split ratio) for balanced detection. The signal collected by a photodetector is digitized with an analog-digital acquisition card and transferred to a computer for processing
In combination with scanning methods, OCT provides three-dimensional information about the scattering properties of living tissue. The spatial resolution that can be reached is near-histologic (micron scale), which makes OCT suitable for in vivo optical biopsy, allowing for the visualization of tissue cross-sectional microstructure even without a contrast agent or dye. Because signals from different depths are separated by the measurement approach, OCT can detect deeper events more easily than a reflection approach. The dependence on the first interaction of photons with the medium imposes maximum penetration limits on the technology that are incompatible with using this approach to study the human brain in a noninvasive manner.
OCT has the potential to provide new imaging, visualization, and quantification capabilities for a wide range of investigations in neuroscience and neuroimaging. Research has demonstrated its potential in the areas of developmental neurobiology, retinal imaging, cellular imaging, brain tumor imaging, and image-guided surgery for the repair of peripheral nerves.20 By incorporating OCT technology into an endoscope Bohringer et al. were able to visualize tissue lining the walls of the third ventricle such as peri-aqueductal tissue, floor of the third ventricle, as well as the rostral wall of the basilar artery in a cadaveric study.24 The same group also analyzed human brain tumor biopsies as well as an orthotopic mouse glioma model using time domain and spectral domain OCT and found good correlation of OCT findings of normal brain, tumor infiltrative brain and tumor with histology.25 OCT has been used to visualize retinal hamartomas in patients with tuberous sclerosis,26 to measure the retinal nerve fiber layer thickness in patients with mild cognitive impairment and Alzheimer’s disease and multiple sclerosis.27,28 Cadaveric studies have shown the potential of OCT for providing navigational guidance of deep brain stimulation probes.29 Recently, the authors of this chapter have used a catheter based OCT system for in vivo cerebrovascular imaging in patients [unpublished].
Neurotherapeutic Applications of LASER and Light
Light Amplification by Stimulated Emission of Radiation (LASER) is monochromatic, coherent and collimated light that when shone into tissue, acts therapeutically, typically by generating heat. Its therapeutic effects rely on the ablative power of photon energy. Laser interaction with tissue has high spatial and temporal selectivity.
Spinal Applications
Laser Discectomy
Minimally invasive techniques have markedly improved the surgical treatment of herniated intervertebral discs and reduced morbidity and hospital stay. Distinct procedures have been developed to perform percutaneous discectomy with the use of lasers.30–33 Choy et al. reported on percutaneous laser discectomies in 12 patients using a Nd:YAG laser introduced through an 18-gauge-needle. Five of these underwent open surgery subsequently. Mayer et al. used a Nd:YAG laser delivered using optical fibers from a flexible endoscope to perform discectomies in six patients.30 Due to lack of an obvious benefit of laser assisted over microsurgical procedures, the clinical use of percutaneous laser discectomy is extremely limited.
Lipomas
Lipomas often wrap around the conus medullaris and nerve roots, and have poorly defined borders with them. The use of lasers in patients with spinal lipomas and lipomeningoceles is to untether the spinal cord and nerve roots from these lesions. The CO2 laser has been quite effective in achieving this through the vaporization of fat. McLone and Maidich reported on 50 cases of pediatric lipomas treated with CO2 laser and found that the use of laser reduced the length of operation, intraoperative blood loss and degree of manipulation of spinal cord and nerve roots.34 They also reported on improved postoperative neurologic function in these patients including recovery from urinary incontinence and improved motor function. Similarly, Maira et al. reported on the efficacy of the CO2 laser to remove large spinal lipomas in two adults patients.35
Intramedullary Tumors
Cerebrovascular Applications
Laser Assisted Vascular Anastomosis
Laser assisted vascular anastomosis was first performed by Yahr and colleagues in the mid 1960s.39 Initially reported on microvessels (0.7–2 mm diameter) this procedure has subsequently been performed on larger vessels (3–8 mm diameter). Jain and Gorisch in 1979 reported on repair of small blood vessels (0.3–1 mm diameter) in the rat using Nd:YAG laser.40 Frazier et al. in 1985, compared end-to-end anastomoses of femoral arteries (1.6 mm diameter) done with CO2 laser versus conventional microvascular suture anastomosis in miniature swine.41 He found that laser anastomosis took less time (20 versus 30 min) and had higher patency rates. In 1986 Quigley et al. reported that intimal hyperplasia was reduced in laser assisted compared with surgical anastomosis.42 He also reported on aneurysm formation in laser anastomosed vessels.43 In 1989, Shapiro et al. reported that following successful end-to-side anastomosis of rat carotid arteries using Nd:YAG laser, 86% remained patent but 23% developed aneurysms in the anastomotic site.44
This high rate of laser induced aneurysm formation and the effectiveness of conventional microsurgical anastomosis have dampened the enthusiasm for clinical use of laser assisted anastomosis in neurosurgery. However since than Tulleken and colleagues have described a novel high flow bypass procedure using an excimer laser to revascularize the ischemic brain that has shown excellent patency without aneurysm formation.45–47 The exact mechanism of laser welding of blood vessels is unclear but has been attributed to denaturation of collagen in the blood vessel walls.41,48 Other potential cerebrovascular applications include use of lasers in reversing cerebral vasospasm.49–51
Neuro-Oncologic Applications
Meningiomas
In 1980, Takizawa et al. reported on the treatment of meningiomas with the CO2 laser.52 Beck described 51 cases of meningiomas treated with Nd:YAG laser, although he did not report the outcome.53 Roux et al. reported on the combination of the CO2 and Nd. YAG laser in treating 17 meningiomas.54 They reported that the combined laser had greater tissue penetration power, with Nd. YAG contributing towards hemostasis. Lombard et al. studied 220 meningiomas reoperated on with laser vs. conventional techniques.55 While patients in both groups improved after surgery, outcome was better in patients with meningiomas in eloquent areas that were treated with laser. Desgeorges et al. studied 164 meningiomas treated with various lasers over a 6 year period and found that complete tumor removal was documented in 83% of cases with 3% mortality.56 They particularly commented on the ability of lasers to remove deep meningiomas that might be difficult to remove with conventional microsurgery.
Additionally, laser treatment had the advantages of reduced brain retraction, small exposures, reduced mechanical manipulation, and decreased intraoperative blood loss. Waidhauser et al. reported on 43 patients with frontobasal meningiomas, treated using Nd:YAG lasers.57 They were able to obtain contact-free shrinkage of tumor, lysis of dural and bony attachments, and coagulation. They opined that Nd:YAG laser assisted surgery facilitated microsurgical dissection and reduced blood loss.
Vestibular Schwannomas
Laser assisted excision of vestibular schwannomas has been previously reported.38,58 Preservation of cranial nerve function is important. Irrigation with suction of the laser plume has been used to minimize thermal injury to structure around the tumor. In 1993, Eiras et al. compared 12 giant vestibular schwannomas excised with CO2 laser to 12 similar tumors operated microsurgically.58 They found that, in their hands, preservation of facial nerve function was better in laser treated patients, although the duration of surgery was longer. Because of poor handling of the tumor-nerve interface and the potential for thermal injury to the nerves the use of lasers is no longer popular in vestibular schwannoma surgery and is more of historic interest.
Pituitary Tumors
In 1982, Takeuchi et al. were the first to report the use of Nd:YAG laser to penetrate the sellar floor during transsphenoidal pituitary surgery.59 Oekler et al. subsequently reported on 15 cases of pituitary adenomas operated similarly, with laser use minimizing capsular bleeding.60 Powers et al. reported using an Argon laser to open the sellar floor, cut through the duramater and vaporize a fibrous prolactinoma.38 Laser use in pituitary surgery is particularly risky due to the possibility of thermal injury to adjacent structures such as the optic nerves, optic chiasm, and the internal carotid artery.
Intra-Axial Brain Tumors
Despite early enthusiasm for the use of lasers for intra-axial brain tumor resection, there remains no clear advantage of this technique over conventional microsurgery. Optical techniques may be more useful for surgical guidance during surgery for intra-axial brain tumors and for photodynamic therapy to the resection cavity for microinvasive disease. Intraoperative measurements have shown that white matter in the brain in distinctly higher in optical scattering of NIR light compared to glioma tissue.61
Laser-induced fluorescence (LIF) spectroscopy and imaging have been used to distinguish neoplastic tissue from normal brain, since normal brain has a characteristic pattern of fluorescence when exposed to light.62,63 This is due to autofluorescence from endogenous fluorophores. Lin et al. combined tissue fluorescence with diffuse reflectance spectroscopy to distinguish normal brain from infiltrating tumor margin and reported a sensitivity and specificity of 100% and 76% respectively.62 Amharref et al. described the use of fourier transform infrared microspectroscopy to distinguish normal brain tissue from tumor and tumor infiltrative brain based on tissue lipid concentrations.64 Leppert et al. described multiphoton excitation to distinguish glioma from normal tissue using autofluorescence decay.65
Fluorescence Guided Resection and Photodynamic Therapy
The extent of surgical resection of gliomas correlates with increased survival.66 However, complete resection of these tumors is often not possible because tumor margins are not readily distinguished from normal brain tissue. In order to further demarcate this, exogenous fluorophores have been administered that preferentially localize in brain tumors. These include Phthalocyanine tetrasulfonate, hematoporphyrin derivative (HPD), and 5-Amino Levulenic Acid (ALA).67–69 Fluorescence guided resection (FGR) of tumors using ALA has been reported to have a sensitivity and specificity of 85% and 100% respectively.70 In an interim analysis of a randomized control trial for FGR of gliomas, Stummer et al. reported complete resection of contrast enhancing tumor in 65% of patients assigned 5-aminolevulinic acid compared with 36% assigned white light.71 Patients allocated 5-aminolevulinic acid had higher 6-month progression free survival than did those allocated white light (41.0% vs. 21.1%), with no difference in short term adverse effects.
Despite the ability of FGR to visualize tumor margins, resection cannot be carried out safely when the tumor has infiltrated brain tissue vital for survival or tissue responsible for important functions (eloquent brain). Photodynamic therapy (PDT) has been attempted to destroy infiltrative tumor cells in tissue.72 In PDT, a photosensitizer is administered systemically that preferentially localizes within tumor cells in microscopically infiltrating tumor tissue. Laser light is then administered into the tissue of interest with spatial localization that excites the photosensitizer, releasing singlet oxygen within tumor cells. Single oxygen being toxic selectively kills tumor cells where it localizes.
The most common photosensitizers used for PDT are HPD and ALA. Clinical studies in patients with recurrent gliomas have demonstrated that PDT can increase survival in these patients and survival appears to correlate with light energy delivered.73,74 The most common cause of PDT treatment failure is local recurrence, which is likely related to limited tissue penetration of light at 630 nm wavelength.75 In general, longer wavelengths of light penetrate the brain better. However since light absorption by HPD and ALA is limited above 630 nm, newer photosensitizers in the future may allow longer activating wavelengths to be delivered possibly improving local control rates.
Benzoporphyrin derivative (BPD) is a newer photosensitizer that can be excited by 680 nm light and thus may have better potential of preventing local recurrence.76 Animal studies with BPD indicated that increased tissue penetration does not result in increased toxicity.76 Encouraging results have been obtained with FGR and PDT in brain tumors. The development of cheaper diode lasers and photosensitizers that absorb light at longer wavelengths for FGR and PDT could potentially improve survival rates from previous studies. Since PDT has been administered in patients only in the acute intraoperative setting, optimal dosing regimen has not been clinically studied. In vitro models have shown that administering PDT in repetitive77,78 and chronic low fluence (metronomic)78,79 doses as well as combinations with ionizing radiation,80 hyperthermia81 and radiosensitizers82 may have superior therapeutic effects compared to single acute PDT treatment alone. Administering repetitive and chronic PDT in patients will require special devices such as indwelling balloon applicators previously described by Hirschberg and colleagues.83
Interstitial Laser Thermotherapy
Hyperthermia has been used as an adjunctive therapy for malignant brain tumors.84,85 The severity of lesions created using laser ablation is proportional to the energy delivered on application time. Interstitial laser thermotherapy using Nd:YAG laser as a thermal source has been studied in animal models and humans.86 Conical sapphire tipped optical fibers delivering a point source of Nd:YAG laser energy can be flexibly directed at any point within a tumor cavity.87–90 Despite initial encouraging results in the treatment of malignant gliomas,85 the role of hyperthermia in clinical neurosurgery is currently limited.
Stereotactic and Functional Neurosurgical Applications
Lasers have been utilized in neurosurgical operations for frameless stereotactic registration. Marmulla et al. described the use of frameless stereotactic laser registration of the auricles for lateral skull base surgery.91 Schicho et al. compared laser surface scanning to fiducial marker-based registration in frameless stereotaxy with comparable results in four cadaveric heads.92 Sinha et al. used laser ranges scanning for intraoperative cortical surface characterization in eight patients with brain tumors and mesial temporal epilepsy.93
In 1986 Kelly et al. described the results of CO2 laser integration into a computer assisted volumetric stereotactic system for brain tumor resection.94 It involved reconstruction of tumor volume from CT and MRI imaging data followed by a computer-monitored stereotactically directed laser to vaporize intracranial tumor. The position of the laser with respect to reformatted planar slices through the tumor was monitored on a computer terminal. In a separate report the same year they reported on 83 computer assisted stereotactic laser procedures in 78 tumor patients.95 Although the authors mention that the stereotactic laser technique was particularly useful in aggressive resection of deep seated tumors within eloquent brain regions, no direct comparison was performed to standard microsurgical techniques. In 1989 Kelly et al. reported on excision of 12 colloid cysts of the third ventricle using stereotactic laser application with excellent results.96
Neuroablative lasers have been utilized in functional neurosurgery particularly in dorsal root entry zone (DREZ) lesions and commissural myelotomies.97–99 DREZ lesions are primarily used for intractable pain following spinal cord injury, brachial plexus avulsions, post herpetic neuralgia, and phantom limb pain.100
Lasers have been used adjunctively in the management of epileptic syndromes. Kelly et al. reported in 18 patients with medically intractable epilepsy that underwent stereotactic amygdalohippocampectomy using a CO2 laser.101 Later they reported on stereotactic laser treatment of epileptogenic lesions associated with tuberous sclerosis.102 While effective, it is unclear whether laser assisted techniques have advantages over conventional microsurgery in the treatment of epileptic lesions.
Neuroendoscopic and Pediatric Applications
Endoscopic applications of lasers in neurosurgery have been mainly in the management of hydrocephalus. Bucholz and Pittman used a Nd:YAG laser to endoscopically coagulate the choroid plexus in an infant with hydrocephalus.103 Vandertop et al. performed reported endoscopic third ventriculostomy with a Nd. YAG laser and a new-generation laser diode in 33 patients and reported no morbidity.104 Powers used an Argon laser to perform endoscopic fenestration in two infants with compartmentalization of the lateral ventricles secondary to ventriculitis.105 Zammorano et al. integrated image-guided stereotaxis with rigid-flexible endoscopy and the Nd-YAG laser (endoscopic laser stereotaxis) and found this system useful in cystic and intraventricular lesions.106 Otsuki et al. used a stereotactic guiding tubes and endoscopes to deliver laser irradiation to deep seated brain tumors.107
Peripheral Nerve Applications
Due to the limitations of microsurgical nerve repair such as scar formation, fascicle mismatching, neuroma formation, inflammatory response to suture material, researchers have been interested in the use of lasers for nerve repair.108 This application is termed “laser neurorrhaphy.”
Laser neurorrhaphy was first reported in 1984 by Almquist et al. with the use of argon laser to repair peripheral nerves in rats and monkeys.109 Fischer et al. reported using CO2 lasers for sciatic nerve repair in rats.110 Seifert and Stoke demonstrated feasibility of microsurgical CO2 laser assisted repair of the oculomotor nerve in cats.111 Bailes et al. compared CO2 laser with 9-0 nylon microsurgical neurorrhaphy in a primate model of sural to peroneal nerve graft and found comparable nerve conduction velocities (NCV) and histologic findings at 5, 8,10 and 12 months.112 Huang et al. compared microsuture technique to CO2 laser repair of sciatic nerves in rats and found the EMG and NCV studies to be comparable113 although Maragh et al. compared laser neurorrhaphy to standard microsurgical nerve repair in rat sciatic nerves and found that nerve conduction velocities were better in the latter group.114 Campion et al. reported improved neuromuscular function of argon laser neurorrhaphy compared to microsurgical epineural surgical repair in rabbits.115 Korff et al. found similar amounts of regeneration in severed rat sciatic nerves repaired with suture or laser, at 2 months.116 Menovsky and Beek compared CO2 laser assisted nerve repair with fibrin glue or absorbable sutures in transected rat sciatic nerves and found no significant differences in motor function at 16 weeks and no histological differences.117
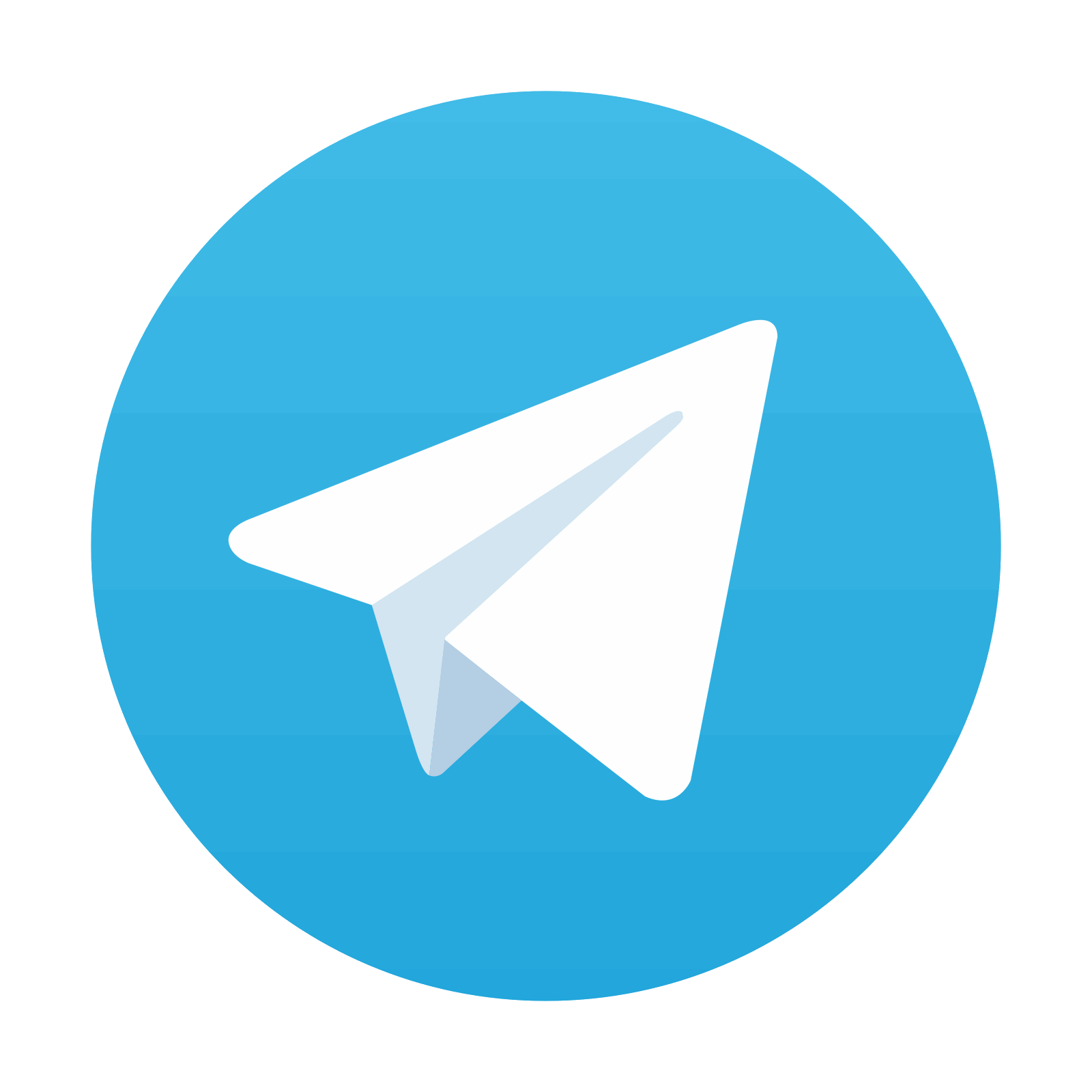
Stay updated, free articles. Join our Telegram channel

Full access? Get Clinical Tree
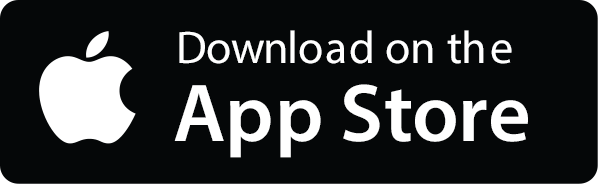
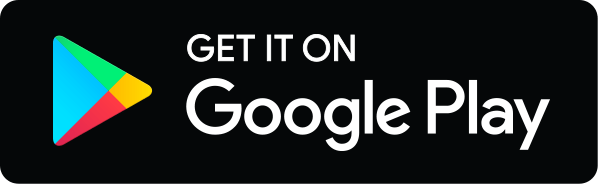