What is next for fractionated laser treatments? New developments on microscopic patterns of injury and sparing using lasers and light sources.
Photochemical tissue bonding (PTB), the next generation of tissue repair?
Selective targeting using photodynamic therapy: development of new photosensitizers and clinical applications.
Low-level light therapy: mechanisms and uses are emerging.
Optical in vivo microscopy: approaching clinical use.
Introduction
In retrospect, it is interesting to realize the way technology and new developments are sometimes so obvious and simple. Looking back over the history of laser surgery, laser tissue ablation (removal) was developed over 40 years. It became popular in the 1980s and 1990s especially because of skin resurfacing, but faded at the end of 1990s due to side effects, and almost vanished from most clinical practices. In 2004, with the introduction of new fractionated methods of skin resurfacing, the same old lasers developed decades ago became popular again, by delivering their beams in microscopic patterns. The technology to make arrays of microscopic laser beams has been available for 40 years, but not used this way in dermatology. The limiting factor is not the technology, but the way we look at it.
Future directions of laser surgery will depend mainly on medical needs and on the creativity of research to meet those needs. Lasers and light sources will probably be involved in developments of tissue engineering, gene therapy, “smart” devices that will be able to detect and treat at the same time, robots that may allow patients to perform safe self-treatment, light activated nanoparticles of selective targeting of cancer cells/infections, and/or combinations of all these and other technologies.
Which recently-developed technologies and strategies are closer to clinical practice? Here, we discuss the future of “fractionated” systems; photochemical tissue bonding, selective targeting using photodynamic therapy, the science behind low-level light therapy and in vivo optical microscopy. However, we do not know what the future holds.
Fractionated Treatments: What Is Next?
The use of microscopic patterns of injury and sparing described by Manstein et al.1 created a new way of skin resurfacing and “photorejuvenation”. Fractionated laser methods stimulate skin remodeling with rapid post-treatment healing, reducing down-time. Therefore, several varieties of “fractionated” systems suddenly became popular offering a number of different options: there are scanning to stamping mode devices, non-ablative and ablative systems. To date, all fractionated laser treatments utilize water as the primary chromophore, with consequent dermal remodeling. However, it is expected that in future, those devices will evolve to “smarter” versions that would be able to destroy specific targets, much as a “smart bomb” is guided to a target.
Biological pathways and responses involved in the fractionated treatments are not fully understood. Skin tightening has been well described,2,3 but it is not known if fractionated treatments can reduce or expand the tissue. Fractional photothermolysis may, or may not, actually rejuvenate skin by forcing renewal and replacement of senescent tissue. Could fractionated treatments be used to treat or expand other solid organs? Could they be used to regenerate neurons, muscle, liver or any other vital cells? Remarkably, up to 50% of skin can be killed or ablated (vaporized) in a treatment session by fractionated treatment, with no apparent scarring or loss of functionality. It is not impossible that fractionated treatments could promote solid organ “rejuvenation”.
Ablative fractional skin treatment may also provide drug delivery (Fig. 1). This has been described in a small pilot study using fractionated non-ablative laser to enhance photodynamic therapy benefits for skin rejuvenation.4 Potentially, fractionated treatment could help treating any deep skin process including: tumors, recalcitrant hyperkeratotic psoriasis, granulomatous diseases, infections and deep mycosis or delivering vaccines.


Fig. 1
Porphyrin fluorescence as a result of fractional ablative CO2 laser-enhanced uptake of topical 20% aminolevulinic acid (ALA). An array of microscopic “laser-created” hole has dramatically increased uptake of ALA (Photo obtained by F.H. Sakamoto, MD, M. Wanner, MD, A.G. Doukas, PhD, W.A. Farinelli, B.S. and R.R. Anderson, MD, Wellman Center for Photomedicine, Massachusetts General Hospital, Harvard Medical School, Boston, MA)
Photochemical Tissue Bonding
Surgical sutures are the most common method for tissue reconstruction and repair. However, both common sutures and staples are not able to prevent fluid leakage, may cause foreign body reactions and scarring, and often require return visits for removal. Synthetic glues and electrical or laser-induced thermal welding may provide immediate tissue bonding but they can also induce scarring or foreign body reactions. Other types of sealants (fibrin and collagen-based) are expensive and not popular among clinicians.
Photochemical tissue bonding (PTB) has recently been created by Irene Kochevar and colleagues using rose-bengal as a photosensitizer and green light for photochemical activation (Fig. 2). The photosensitizer is totally reabsorbed into the body, causes little irritation and has no other known side effects. PTB causes collagen at tissue surfaces to cross-link during about 5 min of light irradiation, yielding a bond with less inflammation or scarring. Preclinical studies for blood vessel, nerve repair,5 cornea,6–8 and skin9,10 have been presented. Interestingly, nerve repair with PTB in combination with human amniotic membrane was shown to be more efficacious than common suture for functional recovery. In comparison to standard sutures, the photochemically sealed amnion wrap also improved histological recovery.5 This novel light-activated method of tissue repair may potentially produce better appearance and functional recovery.


Fig. 2
Comparison of photochemical tissue bonding (PTB) (right) with conventional sutures (left) for superficial closure of skin excision wound. Photo was taken 2 weeks after surgery, immediately after suture removal. Surgery by S. Tsao, MD. PTB technology was developed in the laboratories of I.E. Kochevar and R.W. Redmond, Wellman Center for Photomedicine. Photo by M. Yao, M.D., Ph.D.
Selective Targeting Using Photodynamic Therapy
Photodynamic therapy consist of delivering a light-activated drug or precursor, that after light irradiation reacts with oxygen producing free-radicals such as singlet oxygen and other reactive oxygen species (ROS). The ROS damage certain “targets” in tissue, by inducing apoptosis, necrosis, or activating local inflammatory/immune responses.
Many photosensitizers (PS) are currently available for medical use; in dermatology, psoralens, methylene-blue, aminolevulinic-acid (ALA) and its derivatives, porphyrins and porphyrin-derivatives are the most commonly used and studied for various conditions including actinic keratosis, cancer, vascular mal formations, inflammatory diseases, acne, infections, and more. The potential versatility of PDT is impressive.
The use of light with or without combination of a drug was used even by ancient civilizations in Egypt, India and China to treat skin diseases. Since then, photodynamic therapy (PDT) has become a popular modality of treatment in various systems and organs. However, even with increasing promise and many publications, the clinical impact of PDT has been modest. This is likely to change.
In order to increase efficacy and reduce side-effects, different approaches have been described to improve photosensitizer (PS) delivery and accumulation into targeted structures.11 The development of PS that can penetrate easier into the plasma cell membrane is underway, e.g. by changing molecular physico–chemical properties, with consequent increased and faster local drug accumulation.12 An example is the use of alkyl-esters of aminolevulinic acid (ALA) with higher lipophilicity such as the methyl, propyl and hexyl-esters of ALA12; or the use of liposome-encapsulated molecules, oil-dispersions or polymers.13,14 Some of these new molecules are being studied in pre-clinical studies and in phase I and II clinical trials in the US and Europe.
Another strategy for selective PDT is by changing or modulating the target environment. For example, it is possible to inhibit the accumulation of PS in normal surrounding areas while increasing the amount of PS or its photodynamic effect within the targeted structures. When using ALA and ALA-derivatives to produce PDT, the drug has to be metabolized into porphyrins, especially protoporphyrin IX (PpIX). PpIX is the active PS. PpIX accumulation has been demonstrated to be dependant on heme synthesis enzymes, iron stores, and many other variables. By modulating those variables it is possible to control and produce selective targeting (Fig. 3). Table 1 summarizes different methods to modulate porphyrin-induced PDT that can be used to select tissue targeting.


Fig. 3
Schematic figure representing three phases when porphyrin-induced PDT can be modulated. Phase I: by controlling drug-delivery methods; phase II: by controlling ALA metabolism into porphyrins (the active photosensitizer); phase III: by controlling light and porphyrins interaction during PDT
Table 1
Mechanisms to produce targeted porphyrin-induced photodynamic therapy
1. Modification of ALA formulation: |
(a) Drug concentration |
(b) Delivery vehicle |
(c) Use of liposomes, nanoparticles, polymers, etc. |
(d) Chemically modified lipophilic ALA-derivatives |
2. Modification of target tissue characteristics: |
(c) Use of chemicals to change heme synthesis: hydroxypyridinone (CP94) or desfferioxamine (iron chelators), or others |
(d) Change of molecular oxygen availability in the target tissue at the time of light exposure22 |
3. Modification of ALA/ALA-derivatives and light delivery factors |
(a) Adequate incubation time of the drug in tissue prior to light exposure (time between drug application and light treatment) for each target26 |
(b) Wavelength of light used in treatment |
(c) Adequate light fluence (also called “dose”) in J/cm2 |
(d) Adequate light irradiance (rate at which the fluence is delivered, in W/cm2; 1 W/cm2 = 1 J/cm2/s) |
One example is the use of temperature to control tissue targeting. Since most enzymes are temperature labile, Joe et al. demonstrated local inhibition of PpIX accumulation when temperature is lower than about 20°C, and an increase in PS at temperatures above 37°C.20 Thus, a simple temperature gradient in skin could possibly localize photosensitizer in target tissue and spare normal surrounding areas.
Finally, selective-PDT can be produced by a PS that will actively bind to specific cell-targets, for example, by combining a PS with monoclonal antibodies,27 in a method called photoimmunotargeting (PIT).11 PS can be synthetically conjugated to antibodies or aptamers that deliver the PS by binding to specific molecular markers. Those markers can be located on target cells (e.g. cancer), in cells involved in development of the disease, or in the cells effecting immune response. The use of these “new generation PS” could potentially enhance efficacy and reduce side-effects. However, new drugs often take a long time to reach clinical use.
In general, the simple understanding of the mechanisms of action of PDT and pharmacological aspects of PS can be used for selective targeting: by passive methods, by target modulation or by active targeting using conjugated PS into cell markers. The development of these techniques is promising and will create more efficient treatments.
Low-Level Light Therapy
The use of low-level light therapy (LLLT) has been described for pain treatment, anti-inflammatory effect, wound healing, infections and many other indications, amid certain skepticism among scientists and clinicians. Recently, excellent studies have been published suggesting clinical efficacy and explaining some of the molecular biological mechanisms involved. So far, the exact response pathways are not completely known, but evidence points toward photochemical modification of mitochondrial functions. There are many variables involved such as light wavelength, irradiance, pulsing, and delivered light dose. The understanding of these factors is fundamental for choosing appropriate clinical indications and optimal parameters. It is also important to note that different cell types might respond differently to light.
There are many possible primary chromophores identified in the LLLT process, especially in mitochondria28 (Table 2). Several mitochondrial signaling pathways can be changed such as: redox sensitive pathway, cyclic AMP-dependant signaling, nitric oxide control of respiration and other pathways.28,36
Table 2
Identified LLLT mitochondrial molecular targets and mechanisms of action
Cellular targets | Function | Wavelength | Mechanisms of action |
---|---|---|---|
Cytochrome c oxidase | Cell respiration | Red-NIR | Stimulatory effect29 |
630, 632.78, 650, 660, 725, 820 nm | Increased RNA and protein synthesis30 | ||
Increased proton electrochemical potential and ATP synthesis31 | |||
Photoactive porphyrins | Heme synthesis | Visible | Cell proliferation |
∼410 nm | Tissue stimulation34 | ||
∼500, 540, 585 nm | |||
630 nm | |||
Flavoproteins35 | Initiate free radical reactions | <500 nm | ↓ Oxidative stress |
DNA repair | |||
Apoptosis |
In dermatology, LLLT has been used but not yet well developed or optimized for given applications. Hopefully this will ensure based on great evidence.
In Vivo Optical Microscopy of Skin
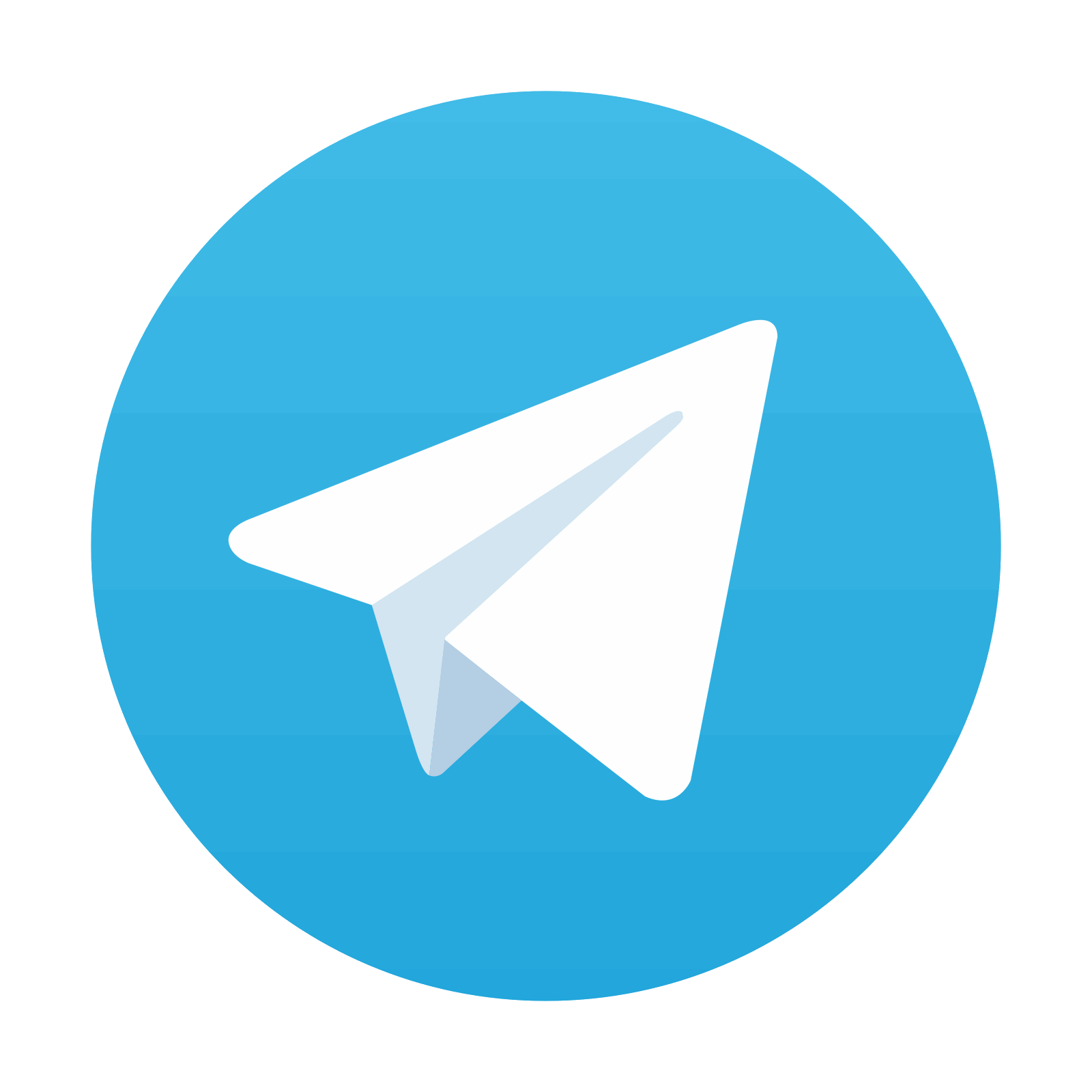
Stay updated, free articles. Join our Telegram channel

Full access? Get Clinical Tree
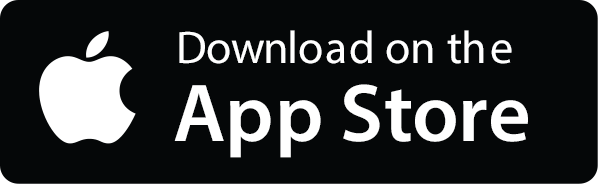
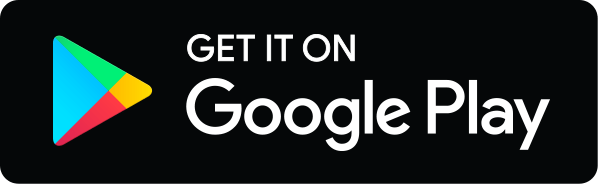