Fig. 12.1
Comparison of cell composition of the BM of the aged microenvironment versus the young microenvironment. Schema depicting the relative content of differentiated cells with recognized effect on HSC activity in young and old microenvironment (primary or transplanted) in a longitudinal section of a long bone
The myeloid skewing and impaired lymphoid differentiation potential observed in aged animals are probably due to both intrinsic (Kim et al. 2003; Dykstra and de Haan 2008) and extrinsic signal changes (Li et al. 2001; Linton and Dorshkind 2004; Donnini et al. 2007; Zhu et al. 2007). The impaired generation of B cells in old mice reconstituted with young donor BM cells and the inefficient secretion of interleukin 7 (IL-7) by stromal cells from old mice indicate that the B lymphopoietic support potential of the hematopoietic microenvironment decreases with age (Stephan et al. 1998; Li et al. 2001).
HSCs have a reduced ability to home to their niches upon transplantation into old recipients compared to young recipients (Liang et al. 2005; Rossi et al. 2005). Modulation of HSC adhesion influences essential cellular processes required for homing and engraftment, a crucial process that allows HSCs to be transplanted in the clinical setting. The essential role of direct cell–cell contact for the regulation of self-renewal and differentiation of adult stem cells has been shown in various organisms and cell systems. Specific junctional complexes may play a similar role in the hematopoietic system. Adhesive interactions of HSCs with their niche are modulated by age-related alterations, as aged mice show an increased mobilization of HSCs in response to granulocyte colony-stimulating factor (G-CSF) (Xing et al. 2006; Ju et al. 2007). Various adhesion proteins have been implicated in this interaction such as N-cadherin (Zhang et al. 2003; Xie et al. 2009; Sugimura et al. 2012), b1/b2-integrin (Zanjani et al. 1999; Papayannopoulou et al. 2001; Priestley et al. 2006; Taniguchi Ishikawa et al. 2013), CD44 and variants (Lewinsohn et al. 1990; Dimitroff et al. 2001; Avigdor et al. 2004; Sackstein et al. 2008; Sackstein 2011), ALCAM (activated leukocyte cell adhesion molecule) (Ohneda et al. 2001; Chitteti et al. 2013), and connexin 43 (Cancelas et al. 2000; Presley et al. 2005; Schajnovitz et al. 2011; Gonzalez-Nieto et al. 2012; Ishikawa and Cancelas 2012; Taniguchi Ishikawa et al. 2012). Of these, losses of ALCAM and connexin 43 have been shown to be associated with HSC aging through microenvironment-dependent effects. Germinal loss of ALCAM results in impaired blood production; myeloid bias; upregulation of aging-associated genes including Selp (Sullivan et al. 2011), Clu (Trougakos et al. 2006), Cdc42 (Florian et al. 2012), and Foxo3 (Renault et al. 2009); and decreased serial repopulation ability of aged long-term HSCs. Connexin 43-deficiency results in an interesting mixed phenotype of bidirectional traffic defect due to microenvironment defects and loss of the replicative potential upon chemotherapy challenge resulting in senescent quiescence of HSCs due to loss of communication with the BM stromal cells (Gonzalez-Nieto et al. 2012; Taniguchi Ishikawa et al. 2012).
Stem cell mobilization is controlled by circadian rhythms (Mendez-Ferrer et al. 2009). This circadian regulation of HSC migration is likely to result in reported HSC immuno-surveillance functions, which affect the adaptive immune system (Massberg et al. 2007) and which is significantly impaired during the aging process (Plowden et al. 2004; Solana et al. 2006). Circadian rhythms of circulation of HSCs depend on central/peripheral nervous system controlled adrenergic innervation of the BM (Mendez-Ferrer et al. 2008; Scheiermann et al. 2012) and neutrophil-mediated clearance of the stem cell niches (Casanova-Acebes et al. 2013). Specifically, short-living neutrophils age in circulation, and during this aging process, they modulate their expression of adhesion and chemokine receptors L-selectin and Cxcr4. Cd62Llow/Cxcr4high neutrophils that have “aged” in the circulation infiltrate the BM and, before they die, promote reductions in the size and function of the hematopoietic niche. Modulation of the niche depends on macrophages and the activation of cholesterol-sensing nuclear receptors. Interestingly, aged BM is relatively enriched in myeloid cells, and therefore, this mechanism may play a role in the aged niche; and the expression of nuclear receptors (including PPARγ2; see above) follows circadian rhythms affecting the metabolism and is amplified by a high-fat diet (Green et al. 2008).
A change in the cytokine profile of the BM occurs during aging. The expression of TGFb in the niche decreases with age (Yamazaki et al. 2009, 2011), and age-associated telomere shortening leads to abnormal cytokine production and a functional decline in mesenchymal progenitor cells, resulting in a reduced capacity of the aged microenvironment to support HSC engraftment and differentiation (Rando 2006). The successive shortening of telomere length with every cell division is one of the best described mechanisms involved in cellular aging. Telomeres are elongated by the enzyme telomerase. Absence of telomerase results in persistent telomere shortening and finally in loss of telomere function, which is associated with replicative senescence or apoptosis. Stem cells normally express telomerase, in contrast to most somatic cells. However, telomerase activity in HSCs is low, and thus, telomere shortening still occurs during aging and limits their proliferative lifespan (Allsopp et al. 2003; Lansdorp 2005). Unfortunately, telomerase overexpression does not result in improved serial HSC engraftment (Allsopp et al. 2003). Furthermore, telomere dysfunction and aging limited the engraftment of transplanted wild-type HSCs. Telomerase dysfunction induces alterations in the BM microenvironment by diminishing the stromal cell compartment and reducing the capacity of the BM and thymic stromal cells in their hematopoiesis-supportive activity (Ju and Rudolph 2006), and in systemic alterations that result in impaired B-and T-cell lymphopoiesis (Song et al. 2010). Terc-deficiency induced an age-dependent dysfunctional environment that correlated with progressive telomere shortening and limited the engraftment of transplanted wild-type HSC (Ju et al. 2007). These results provide clear evidence that telomere shortening is neither the unique nor an exclusively intrinsic mechanism for cellular aging in HSCs. In contrast, the loss of telomeres induces age-dependent alterations in the stem cell environment that can impair the function and engraftment of HSCs.
Aging of the microenvironment influences clonality in hematopoiesis. Clonality of identical pools of transduced HSCs exposed to a young or aged microenvironment in vivo is primarily oligoclonal within a young microenvironment, while in aged animals the transduced HSCs displayed reduced clonality (Vas et al. 2012a), providing a basis to explain a predisposition to clonal expansion in aged hematopoiesis as a putative substrate for transformation and leukemogenesis.
Aging of HSCs might also be attributed to accumulation of by-products that upon chemical modification of cellular proteins results in continuous accumulation of cellular defects. Two examples of these posttranslational modifications resulting in HSC aging have been well documented. Glycation of proteins leads to formation of cross-linked proteins (Finkel and Holbrook 2000). In HSCs, protein glycation has been demonstrated during aging and is expected to act through extrinsic and intrinsic pathways (Baraibar and Friguet 2013). Reactive oxygen species (ROS) arise as by-products of numerous metabolic processes and cause direct damage to proteins, membranes, and DNA, resulting in a vicious cycle, since accumulation of endogenous DNA mutations contributes to aging of HSCs (Kenyon and Gerson 2007). It has been shown that ROS can regulate HSC function in a concentration-dependent manner. Over time, these oxidant-damaged proteins and DNA accumulate and eventually disrupt cellular function. High levels of ROS can induce HSC senescence and apoptosis secondary to DNA damage (Ito et al. 2006). There is experimental evidence that regionally defined functional hypoxia plays a role in regulating the mesenchymal microenvironment (Fehrer et al. 2007) and cells isolated from distinct BM locations have been associated with local hypoxic gradients (Parmar et al. 2007). However, in vivo analysis of oxygen distribution in the BM has not shown distinctly hypoxic regional areas (Nombela-Arrieta et al. 2013) suggesting that HSCs are subject to similar oxygen tensions as other cells in the BM. Recently, a novel molecular pathway of hematopoietic microenvironment-dependent ROS scavenging has been reported. The integrity of this pathway is required to prevent HSC senescence. The loss connexin 43 function results in the loss of BM stromal-mediated ROS scavenging and subsequent premature senescence and/or apoptosis (Taniguchi Ishikawa et al. 2012).
Epigenetic mechanisms such as DNA methylation or histone acetylation regulate gene transcription at the chromatin level. As these modifications can be inherited by daughter cells, they have been demonstrated to play key roles in altering the long-term fate of HSCs, and hence, they might also be involved in the regulation of intrinsic HSCs (De Haan and Gerrits 2007) or microenvironment aging including MSC aging (Li et al. 2011). Gene expression analysis of HSCs from old mice compared with those from young mice revealed that genes involved in chromatin remodeling and transcriptional silencing were downregulated. Moreover, old HSCs showed transcriptional upregulation of genes involved in inflammatory response and stress, suggesting epigenetic changes, and susceptibility to modification by microenvironment signals (Chambers et al. 2007). Wagner et al. suggests that the hematopoietic microenvironment may condition multiple transcriptional signals since a large number of genes were differentially expressed in human HSC/P upon culture with stromal cells, which affected basic cell functions including cell division, reorganization of the cytoskeleton, and genetic stability (Wagner et al. 2007). The same group found a set of non-translated microRNAs implicated in the process of posttranscriptional gene expression and replicative senescence of MSCs (Wagner et al. 2008). Examples of epigenetic regulation of microenvironment aging include microRNA-dependent regulation and methyltransferase activity regulation. For instance, microRNA-34a, a known tumor suppressor that regulates silent information regulator 1 (Sirt1) expression that was shown to induce senescence in endothelial progenitors and impair angiogenesis (Zhao et al. 2010) and transcriptional expression of DNA (cytosine-5)-methyltransferase 1 (Dnmt1) isoforms, is highly upregulated upon contact between human HSC/P and BM stromal cells (Wagner et al. 2005).
12.3 Conclusion
There is clear evidence of the effects of the aging microenvironment as manifested in modified production of cytokines, chemokines, and signaling pathways that affect HSC senescence, migration, or differentiation. The remaining question is whether different HSC populations respond differently to microenvironment aging. Clonal analysis followed by expression analysis, metabolic profiling, and functional assays will provide us with the information on the crucial mechanisms responsible for microenvironment-dependent HSC aging. Results from this research are expected to provide novel therapeutic targets for intervention in the context of aging and cancer predisposition.
References
Allsopp RC, Morin GB et al (2003) Telomerase is required to slow telomere shortening and extend replicative lifespan of HSCs during serial transplantation. Blood 102(2):517–520PubMed
Ara T, Tokoyoda K et al (2003) Long-term hematopoietic stem cells require stromal cell-derived factor-1 for colonizing bone marrow during ontogeny. Immunity 19(2):257–267PubMed
Arai F, Suda T (2007) Regulation of hematopoietic stem cells in the osteoblastic niche. Adv Exp Med Biol 602:61–67PubMed
Avigdor A, Goichberg P et al (2004) CD44 and hyaluronic acid cooperate with SDF-1 in the trafficking of human CD34+ stem/progenitor cells to bone marrow. Blood 103(8):2981–2989PubMed
Baraibar MA, Friguet B (2013) Oxidative proteome modifications target specific cellular pathways during oxidative stress, cellular senescence and aging. Exp Gerontol 48(7):620–625PubMed
Calvi LM, Adams GB et al (2003) Osteoblastic cells regulate the haematopoietic stem cell niche. Nature 425(6960):841–846PubMed
Cancelas JA, Koevoet WL et al (2000) Connexin-43 gap junctions are involved in multiconnexin-expressing stromal support of hemopoietic progenitors and stem cells. Blood 96(2):498–505PubMed
Cao JJ, Kurimoto P et al (2007) Aging impairs IGF-I receptor activation and induces skeletal resistance to IGF-I. J Bone Miner Res 22(8):1271–1279PubMed
Casanova-Acebes M, Pitaval C et al (2013) Rhythmic modulation of the hematopoietic niche through neutrophil clearance. Cell 153(5):1025–1035PubMedCentralPubMed
Chambers SM, Shaw CA et al (2007) Aging hematopoietic stem cells decline in function and exhibit epigenetic dysregulation. PLoS Biol 5(8), e201PubMedCentralPubMed
Chitteti BR, Cheng YH et al (2013) Hierarchical organization of osteoblasts reveals the significant role of CD166 in hematopoietic stem cell maintenance and function. Bone 54(1):58–67PubMedCentralPubMed
Chow A, Lucas D et al (2011) Bone marrow CD169+ macrophages promote the retention of hematopoietic stem and progenitor cells in the mesenchymal stem cell niche. J Exp Med 208(2):261–271PubMedCentralPubMed
De Haan G, Gerrits A (2007) Epigenetic control of hematopoietic stem cell aging the case of Ezh2. Ann N Y Acad Sci 1106:233–239PubMed
Dimitroff CJ, Lee JY et al (2001) CD44 is a major E-selectin ligand on human hematopoietic progenitor cells. J Cell Biol 153(6):1277–1286PubMedCentralPubMed
Ding L, Morrison SJ (2013) Haematopoietic stem cells and early lymphoid progenitors occupy distinct bone marrow niches. Nature 495(7440):231–235PubMedCentralPubMed
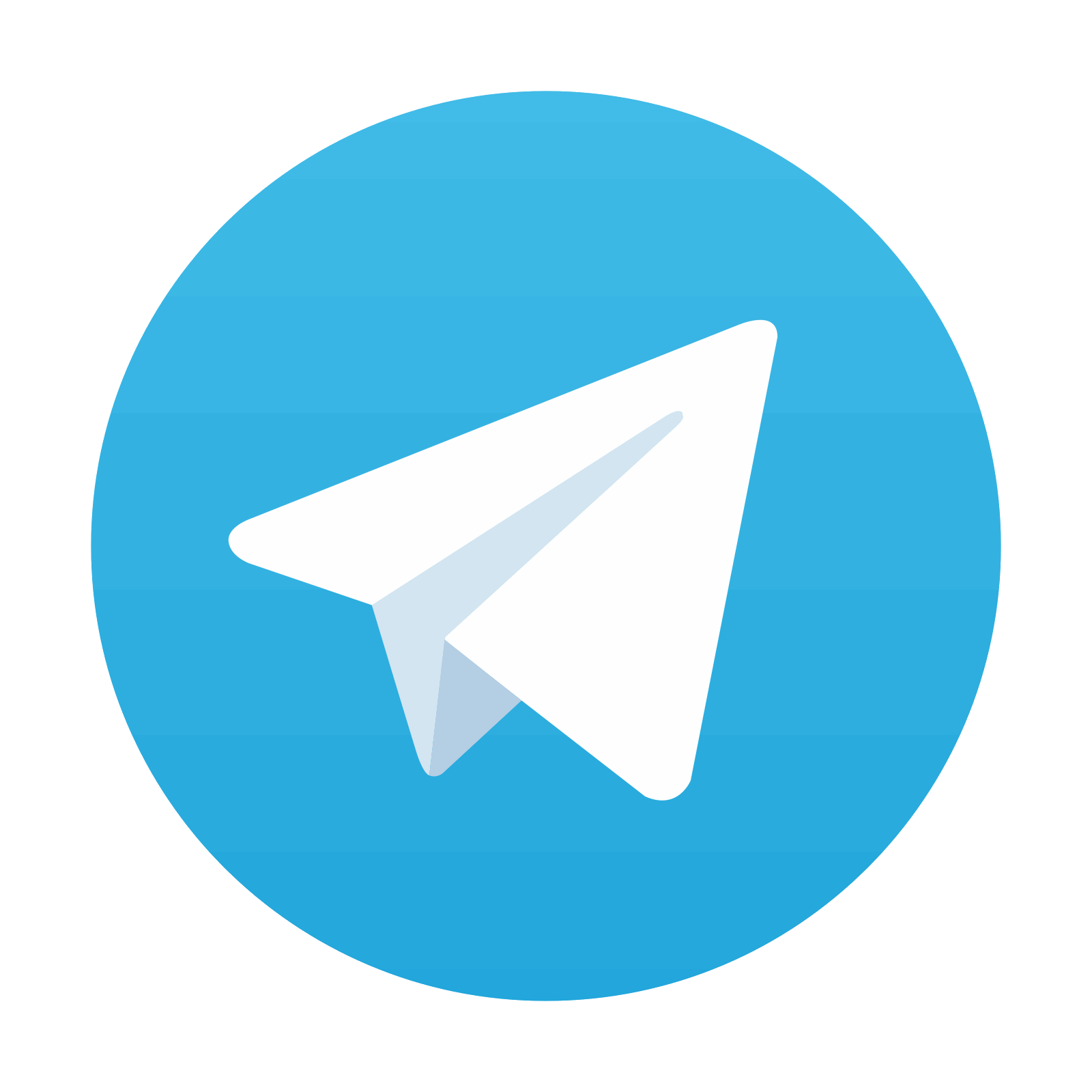
Stay updated, free articles. Join our Telegram channel

Full access? Get Clinical Tree
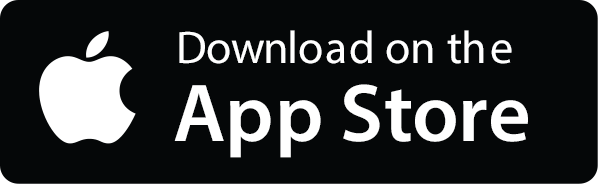
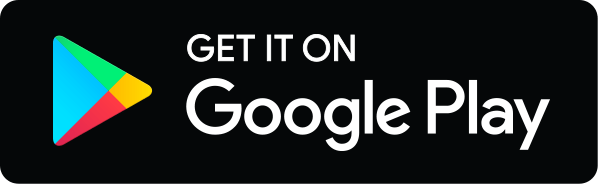