Fig. 5.1
Stem cell populations in the Drosophila m. digestive tract
5.2.1 Cardia and Stomach
Multipotent stem cells (GaSCs) have been identified in the cardia (also called proventriculus – Fig. 5.1), a structure that connects the crop, the esophagus, and the anterior midgut (Singh et al. 2011). These progenitors were found to continuously give rise to cells that compose the cardia itself as well as the crop, under unstressed conditions, suggesting that they are multipotent and constantly proliferate.
The central section of the fly midgut generates an acidic environment, analogous to the mammalian stomach (Shanbhag and Tripathi 2009). The epithelium of this region, including the copper cells responsible for the acidification, interstitial cells, and endocrine cells, is maintained by a specialized population of multipotent gastric stem cells (GSSC – Fig. 5.1) (Strand and Micchelli 2011). These cells are relatively quiescent but rapidly proliferate in response to specific tissue damage. The induction of GSSC proliferation by stress suggests that the behavior of this cell population may change over time. However, potential age-related changes in these tissues and effect of aging on the GaSC and GSSC populations remain to be tested.
5.2.2 Malpighian Tubules
Similar to the kidneys in mammals, the excretory function in Drosophila is fulfilled by specialized organs, the Malpighian tubules. It has been recently recognized that this tissue is maintained by a multipotent stem cell population, the renal and nephric stem cells (RNSCs – Fig. 5.1) (Singh et al. 2007). These cells are capable of self-renewal and give rise to progenitors that can differentiate in all the cell types that compose the tubule (i.e., renalcytes, principal cells, and stellate cells). The mechanisms that control SC proliferation and differentiation in this lineage are starting to be elucidated (Singh et al. 2007; Zeng et al. 2010). However, the impact of aging on the maintenance and turnover of this tissue remains unknown.
5.2.3 Hindgut
The most posterior portion of the intestine is formed by the hindgut. Recently, proliferating cells have been identified in the most anterior segment of the hindgut, the hindgut proliferation zone (HPZ) (Takashima et al. 2008). The anterior portion of the HPZ is composed of a stem cell population, the spindle cell zone (SCZ), which maintains the hindgut epithelium (Fig. 5.1). Contrary to all other stem cell lineages identified in the adult fly digestive tract, these stem cells give rise to a clearly identified transit-amplifying progenitor population.
While these cells were first described as continuously proliferating (Takashima et al. 2008), additional studies have demonstrated that the SCZ stem cell population is mostly quiescent in healthy young animals (Fox and Spradling 2009). Interestingly, proliferation of stem cells and transit-amplifying cells is dramatically increased in response to tissue damage (Fox and Spradling 2009). As the aging intestine is characterized by a chronic exposure to a damaging environment (see below), it is expected that aging can affect the proliferative behavior of these hindgut progenitor cells. In addition, the Drosophila hindgut has recently been described as a model of infection- and oncogene-mediated cell dissemination and metastasis (Bangi et al. 2012; Christofi and Apidianakis 2012). However, this process remains to be investigated in the context of the aging hindgut epithelium.
5.2.4 Posterior and Anterior Midgut
The intestinal stem cells (ISCs – Fig. 5.1) population, present in the posterior and anterior midgut, was the first multipotent stem cell population described in the digestive tract (Micchelli and Perrimon 2006; Ohlstein and Spradling 2006). ISCs were first identified as small diploid cells interspaced between the large absorptive cells that compose most of the epithelium (enterocytes, ECs) and located in close contact with the basal membrane (Baumann 2001). However, only recent lineage tracing experiments have demonstrated that ISCs continuously proliferate and maintain the intestinal epithelium by producing new ECs and enteroendocrine cells (EEs) (Micchelli and Perrimon 2006; Ohlstein and Spradling 2006). Following the discovery of ISCs, the Drosophila midgut has rapidly emerged as an ideal model system to study the mechanisms that control intestinal homeostasis and all aspects of tissue-specific stem cells, under unchallenged conditions or during tissue repair (Biteau et al. 2011; Jiang and Edgar 2012; Wang and Hou 2010).
Interestingly, ISCs continuously proliferate at low rate, replacing the entire epithelium several times throughout the life of the animal, in particular in females that have a much higher nutritional demand to accommodate egg production (Jiang et al. 2009). Normal tissue turnover requires a variety of signals that control ISC self-renewal, proliferation, and differentiation, including the following pathways:
In addition, cell proliferation is rapidly induced in the intestine in response to stress and infection, in order to replace damaged differentiated cells and maintain the integrity of the epithelium. This proliferative response directly involves the activation of ISCs themselves, as no transit-amplifying cell population exists in the midgut, and relies on the induction of many conserved signaling pathways, such as:
While the impact of aging on RNSCs, GaSCs, GSSCs, and the SCZ remains to be investigated, the age-related changes that impair ISC function in older flies are starting to be elucidated. These mechanisms include extrinsic and intrinsic signals, which are largely related to the chronic inflammatory state that develops in the aging intestinal epithelium, and cause uncontrolled ISC proliferation and differentiation defects. In addition, this stem cell population has proven to be a valuable model to address critical questions regarding the influence of stem cell-mediated tissue homeostasis on physiology and longevity.
5.3 Age-Related ISC Hyper-proliferation
ISCs were first characterized as a slow cycling stem cell population. Only a few cells, out of the thousands of ISCs dispersed in the midgut, are undergoing mitosis at any given time in young animals. However, ISC proliferation dramatically increases with age (Biteau et al. 2008; Choi et al. 2008). This hyper-proliferation is reminiscent of the response to tissue damage, which relies on an increased ISC proliferation to replace damaged differentiated cells (Amcheslavsky et al. 2009; Buchon et al. 2009b; Choi et al. 2008; Cronin et al. 2009; Jiang et al. 2009). This insight led to the current model that proposes that the chronic activation of the mechanisms that control tissue repair is the primary cause of the changes observed in the aging intestine and age-related disruption of the ISC function.
The aging process in the posterior midgut includes changes in the intestinal environment, in particular in the gut microbial flora, and the activation of both non-cell-autonomous and intrinsic signals that ultimately drive uncontrolled ISC proliferation (Fig. 5.2). However, many questions remain unanswered. For example, the mechanisms that contribute to the changes in bacteria population are still not fully understood; the specific contribution of each signaling pathway remains unclear; and it has yet to be tested whether some aspects of the intestinal and ISC aging are independent of the gut flora and stress responses and directly related to an intrinsic aging process in ISCs.
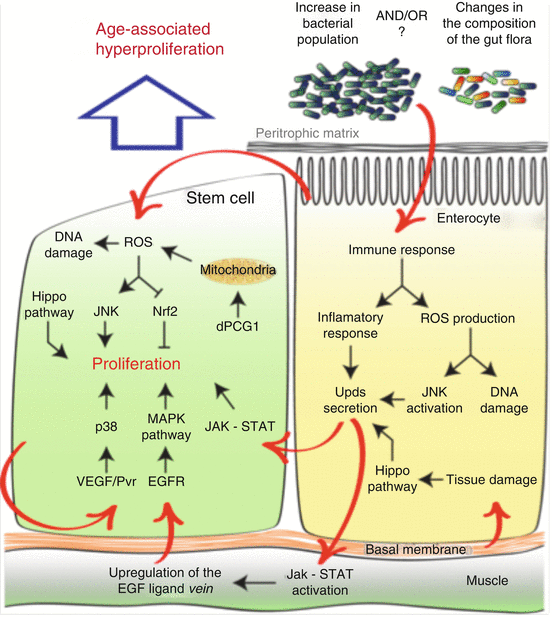
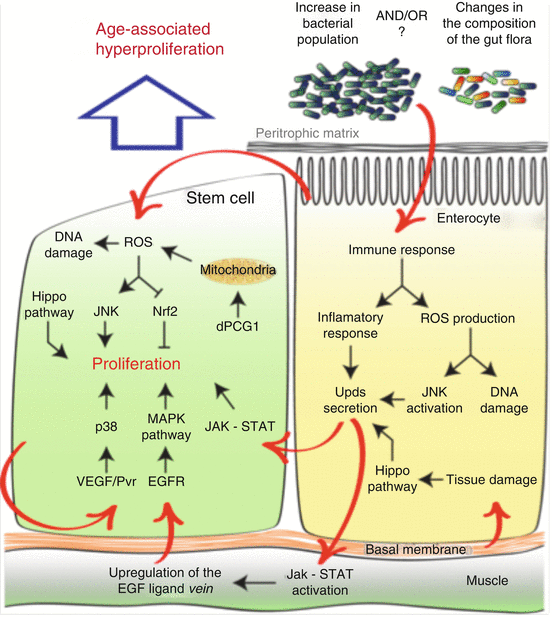
Fig. 5.2
Schematic representation of the numerous cell-autonomous mechanisms and extrinsic signals that may contribute to the ISC hyper-proliferation observed in the aging Drosophila intestine
5.4 Environmental Changes and Extrinsic Signals
5.4.1 Role of Age-Related Changes in the Intestinal Flora
Like the intestine in other animals, the Drosophila midgut hosts a variety of commensal, food-borne, and pathogenic bacterial populations (Hooper and Gordon 2001; Sansonetti 2004). Flies have evolved a variety of mechanisms to accommodate beneficial flora and defend the intestinal epithelium against pathogens (Ryu et al. 2010). Interestingly, dramatic changes in the intestinal flora occur during aging in mammals (Tiihonen et al. 2010; Woodmansey 2007). Supporting the notion that similar changes in gut flora strongly contribute to the aging process of the intestinal epithelium in Drosophila, maintaining flies in an axenic environment prevents age-induced ISC proliferation and intestinal dysplasia (Buchon et al. 2009a). However, it is still lacking a clear description of age-related changes in the composition and/or size of bacterial populations in the midgut of older flies. In addition, the reasons for these potential changes remain unclear. Several non-exclusive mechanisms may drive these alterations, such as defects in the immune system (i.e., immunosenescence); decay of the peritrophic membrane, a structure essential to protect the intestinal epithelium against bacterial infections (Kuraishi et al. 2011); changes in intestinal function and chemical properties; and selection or expansion of more virulent bacterial strains over time. Importantly, two recent studies have started to address this question and suggest that a collapse of proper immune signaling in the gut itself and the adipose tissue, another essential immune organ in flies, plays a fundamental role in dysbiotic changes occurring in older animals (Chen et al. 2014; Guo et al. 2014). Yet, further investigations will be required to test all the possible factors contributing to age-related changes in microbiota and fully understand the underlying age-related molecular changes.
5.4.2 Bacterial Infection and Tissue Damage: The ISC Response to Enterocyte- and Muscle-Derived Proliferative Signals
After establishing that bacteria that populate the intestine play a major role in the age-related degeneration of this tissue, one essential question emerges: how do changes in the gut flora influence intestinal homeostasis and ISC proliferation in older flies? Answers to this question came from the study of the mechanisms that control intestinal regeneration in response to bacterial infection and tissue damage (Fig. 5.2).
Production of reactive oxygen species (ROS) is one of the major responses of the intestinal epithelium to cope with bacterial infection, and specific mechanisms to fight against this oxidative stress have been identified in Drosophila (Ha et al. 2009, 2005a, b). Interestingly, antioxidant molecules, such as N-acetylcysteine and glutathione, prevent induction of ISC proliferation after bacterial infection (Buchon et al. 2009a), while exposure to ROS-generating molecules dramatically increases ISC proliferation (Biteau et al. 2008; Choi et al. 2008), indicating that this oxidative burst directly impacts ISC proliferation.
The increased ROS production and the recognition of bacteria themselves lead to the stimulation of multiple signaling pathways in ECs and the establishment of both an antimicrobial and an inflammatory response, which are essential for the survival of the organism (Buchon et al. 2009a, b; Cronin et al. 2009; Jiang et al. 2009). One major component of this response is the activation of the JNK signaling pathway in ECs, which induces the production of interleukin-like cytokines (unpaired, Upds). This secretion of Upds by ECs leads to the activation of the JAK-STAT signaling pathway in ISCs, directly promoting their proliferation (Buchon et al. 2009a, b; Jiang et al. 2009). At the same time, this inflammatory signal causes the activation of JAK-STAT in the visceral muscle that surrounds the intestinal epithelium. This results in the upregulation of the expression of the EGF ligand vein, which in turn further promotes stem cell proliferation through the activation of the EGFR-MAPK signaling pathway in ISCs (Buchon et al. 2010; Jiang et al. 2011).
Similar to the response to bacterial infection, damage to the intestinal epithelium, such as DNA damage or disruption of the basal membrane, promotes ISC proliferation and tissue repair through the activation of the same stress-responsive signaling pathways (Amcheslavsky et al. 2009).
Over the last few years, it has become clear that during normal aging, similar mechanisms are chronically activated in the intestine. For example, high levels of ROS and increased activity of the JNK pathway are detected in ECs of older flies (Biteau et al. 2008; Hochmuth et al. 2011), and the expression of proliferative signals such as EGF ligand and Upd cytokines is greatly elevated in the aging fly midgut. In addition, the artificial activation of the mechanisms that promote ISC proliferation in response to infection or tissue damage recapitulates the dysplastic phenotype observed in old animals. Finally, recent evidence suggests that the muscles that surround the gut epithelium deteriorate with age (Larson et al. 2012), a process that may indirectly affect ISCs, through the deregulation of muscle-derived proliferative signals (in particular the ligand vein).
Taken together, these studies strongly suggest that chronic activation of the non-cell-autonomous mechanisms that control tissue repair in young animals significantly contributes to the uncontrolled ISC proliferation and intestinal dysplasia observed in older flies. However, it is important to note that the current model remains to be conclusively validated, as the effects of stress signaling pathways in ECs on age-related changes in the ISC regulation and intestinal homeostasis have yet to be directly investigated.
5.4.3 Other Cell-Cell and Tissue-Tissue Interactions
Interestingly, other cell types and tissues have been shown to interact with ISCs and the midgut epithelium to control stem cell proliferation and the function of the intestine. While little is known about the potential effect of aging on these other interactions, it is important to take these into account when trying to elucidate the complex mechanisms that affect ISC in aging flies, as defects in these mechanisms may directly contribute to the ISC hyper-proliferation and differentiation defects observed in the intestine of old flies.
Within the intestinal epithelium, all the different cell types constantly communicate to maintain homeostasis. Undifferentiated EC-committed daughter cells (i.e., enteroblasts, EBs) are capable of repressing ISC proliferation through the maintenance of E-cadherin-mediated cell-cell interactions (Choi et al. 2011). This interaction is directly regulated by the activity of the insulin receptor pathway in EBs, a conserved signaling pathway that is strongly influenced by aging (see below). At the same time, secretory cells (i.e., enteroendocrine cells, EEs) indirectly control ISC proliferation by regulating muscle-derived production of dilp3 (insulin) and vein (EGF) (Amcheslavsky et al. 2014; Scopelliti et al. 2014). Hence, while age-related defects in these feedback mechanisms have not yet been investigated, impaired signaling in EBs and/or EEs may significantly contribute to the uncontrolled proliferation of aging ISCs.
The complex interactions between ISCs, ECs, and the surrounding visceral muscle were described before. In addition to this regulatory network, other neighboring tissues influence the cells of the intestinal epithelium. The tracheal system, which delivers oxygen to all tissues in the fly, also provides an essential factor that promotes EC survival (Li et al. 2013). Loss of this signal, dpp (decapentaplegic, a member of the bone morphogenetic protein family), induces a strong compensatory ISC proliferation. In addition, the intestine is innervated by a complex neuronal network. These neurons control the adaptation of the intestinal function and excretion to nutrient availability and reproduction (Cognigni et al. 2011). Age-related changes in these organs have not yet been identified, but it is conceivable that misregulation of the signals originating from these tissues participates to the decline in intestinal function in older animals.
Finally, ISCs are regulated by systemic factors, including the activity of the insulin signaling pathway and nutrient availability. In Drosophila, insulin-like peptides (dilps) are secreted by multiple tissues. Notably, dilp2, dilp3, and dilp5 are produced by the neurosecretory insulin-producing cells (IPCs) in the brain (Teleman 2010). Relevant to the regulation of ISCs, dilp3 is also expressed in the visceral muscle surrounding the intestine and regulates the proliferation and number of ISCs in the intestine in response to changing nutritional conditions (O’Brien et al. 2011). Importantly, modulation of the Insulin signaling pathways is the one of the most widely conserved mechanisms that affects aging and longevity, from worm to mammals (Fontana et al. 2010; Kenyon 2010; Partridge et al. 2011). Indeed, reducing insulin signaling systematically or specifically in ISCs prevents age-related intestinal dysplasia (Biteau et al. 2010). Similarly, changes in nutrient availability that are known to affect insulin signaling, aging, and longevity modulate ISC proliferation (McLeod et al. 2010). These observations thus support the notion that, in Drosophila, age-related changes in metabolism and insulin signaling impact the biology of ISCs and that alterations of insulin signaling influence aging and extend lifespan, at least in part, by controlling the function of the stem cell pool in the intestine in old flies.
5.5 Cell Intrinsic Induction of ISC Proliferation
In addition to signals received from ECs and other surrounding cells and organs in response to tissue damage and infection, ISCs are capable of directly sensing changes in their environment and intracellular state and adapt their proliferation rate to these challenging conditions. As a consequence, cell-intrinsic mechanisms also significantly contribute to the changes in ISC regulation that take place in the intestine of aging flies.
5.5.1 Redox State and Stress Signals in Aging ISCs
Supporting the notion that ISC themselves are stressed in the aging intestine, high levels of ROS are detected in old ISCs (Hochmuth et al. 2011), and ISCs accumulate damaged macromolecules with age, as shown by the activation of the DNA damage response (γH2AX) and presence of DNA lesions (8-oxo-deoxyguanosine) (Park et al. 2012). Furthermore, overexpression of antioxidant proteins (the peroxiredoxin Jafrac1 and Gclc, an enzyme in the glutathione biosynthetic pathway) in ISCs and EBs is sufficient to reduce ISC proliferation and delay the progression of the intestinal dysplastic phenotype (Biteau et al. 2010; Hochmuth et al. 2011). These intracellular prooxidant conditions lead to the cell-autonomous activation of multiple signaling pathways that promote ISC proliferation, including the JNK and MAPK p38 pathways (Biteau et al. 2008; Park et al. 2009). At the same time, the Nrf2 signaling pathway, which promotes ISC quiescence under normal conditions, is inactivated, further increasing ISC proliferation rate (Hochmuth et al. 2011). Importantly, genetic modulations of these signaling pathways that revert the influence of aging on their activity are sufficient to prevent age-related tissue degeneration in the posterior midgut (Biteau et al. 2010; Hochmuth et al. 2011; Park et al. 2009), demonstrating the critical role of the cell-autonomous activation of stress signaling in the aging of the intestinal stem cell population.
In addition to the response to oxidative stress, ISCs are capable of responding directly to changes in the epithelium integrity and cell-cell interactions, through the inactivation of the Hippo pathway. Decreased activity of this pathway in ISCs leads to the activation of the transcription factor Yorkie and results in increased ISC proliferation (Ren et al. 2010; Shaw et al. 2010). Therefore, age-related changes in the architecture of the intestinal epithelium are likely to lead to the activation of Yorkie and also participate to the establishment of a chronic ISC-proliferative state.
5.5.2 Role of Mitochondrial Function in ISC Aging
As discussed previously, the increasingly prooxidant ISC microenvironment and the intracellular redox imbalance appear to be the major causes of the increased proliferation of aging ISCs. In addition to the ROS produced by ECs as part of the immune response, it has recently been recognized that mitochondria in aging ISCs themselves are an additional source of ROS (Rera et al. 2011). This recent study suggests that the mitochondrial function in the intestine declines with age. Importantly, reverting these effects of age by increasing the expression of the fly homolog of the PGC1 transcription factors is sufficient to promote mitochondrial activity, decrease the accumulation of ROS in aging ISCs, and prevent ISC hyper-proliferation. These recent findings further confirm that age-related intracellular changes in ISCs directly affect their function in older animals.
5.6 Age-Associated Defects in the ISC Lineage
In mammalian organs, age-related changes in the proliferative behavior of stem/progenitor cells are often accompanied by defects in the mechanisms that control cell-fate decision and differentiation in aging tissues (Brack and Rando 2007; Kim et al. 2012; Rossi et al. 2008). This can generate an imbalance between the different cell types that compose the tissue and/or lead to the production of nonfunctional cells, significantly contributing to age-related loss of tissue function. Similarly, in the aging Drosophila intestine, ISC hyper-proliferation results in a defective lineage that produces large numbers of abnormal cells (Biteau et al. 2008). Therefore, a better understanding of the mechanisms that control ISC self-renewal and cell-fate decision in young animals is essential to fully elucidate the mechanisms that drive dysplasia in the intestine of older flies.
5.6.1 The Posterior Midgut Lineage: Description of the Lineage
Lineage tracing analyses and the use of many cell-specific markers have allowed the elucidation of the ISC lineage in Drosophila posterior midgut (Fig. 5.3). These multipotent stem cells were first described as generating at least three different cell types, nonproliferating undifferentiated progenitors (enteroblasts, EBs), which differentiate in either enterocytes (ECs) or enteroendocrine cells (EEs) (Micchelli and Perrimon 2006; Ohlstein and Spradling 2006). However, it has recently been recognized that the ISC lineage is much more complex than what was originally thought. ISCs primarily asymmetrically divide to give rise to another ISC and a daughter that will undergo differentiation. Yet, ISCs have also been shown to symmetrically divide and generate two ISCs or two daughter cells (de Navascues et al. 2012; Goulas et al. 2012; O’Brien et al. 2011). In addition, it has recently been found that ISCs can give rise to two distinct types of daughter cells: EBs, committed to the absorptive lineage (ECs), and pre-EEs, which differentiate into secretory cells (Biteau and Jasper 2014; Zeng and Hou 2015).
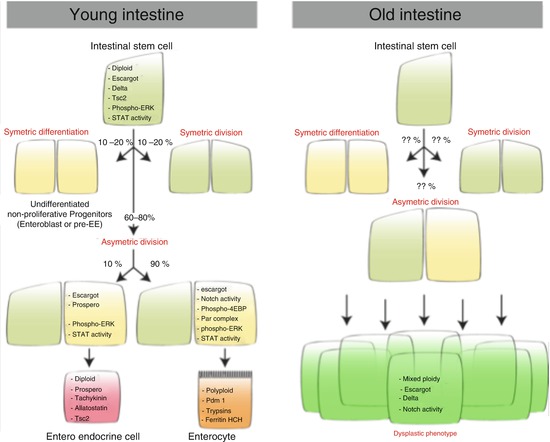
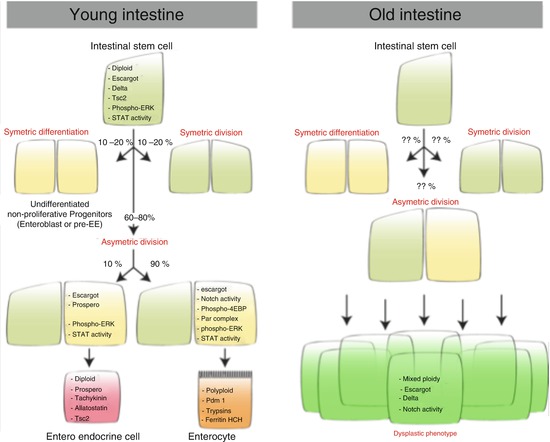
Fig. 5.3
The ISC lineage in young and old flies. Molecular markers of each cell type that composes the intestinal epithelium are indicated
5.6.2 Molecular Mechanisms Controlling the ISC Lineage
While the intestinal lineage is relatively well characterized, the mechanisms that regulate the balance between self-renewal and differentiation remain largely unclear. In this regard, ISC seems to share some similarities with larval neuroblasts, as suggested by the asymmetric segregation of some components of the Par complex (Goulas et al. 2012), which may specify the EB cell fate during cell division. In addition, it has been suggested that the wingless/Wnt signaling pathway is required for ISC maintenance and may control self-renewal (Lin et al. 2008). Also, the balance between self-renewal and differentiation can be dynamically regulated, in particular during the regrowth of the intestine in response to re-feeding after a period of starvation, a process that may involve the insulin signaling pathway (O’Brien et al. 2011). Several models have been developed to describe the mechanisms that control the decision between self-renewal and differentiation (de Navascues et al. 2012; Kuwamura et al. 2012; Perdigoto et al. 2011). However, it has been recently found that endocrine cells directly control their own production, through the Slit/Robo2 signaling pathway, ensuring that Prospero-expressing daughter cells, which are committed to the secretory lineage, are only produced when EEs are missing in the immediate ISC vicinity (Biteau and Jasper 2014).
In addition to self-renewal signals, multiple conserved signaling pathways have been shown to be critical for the differentiation of ISC daughter cells. Notably, the Notch signaling pathway is absolutely required for the differentiation of EBs into ECs, while the JAK/STAT pathway is involved in the differentiation of EEs (Beebe et al. 2010; Lin et al. 2010; Ohlstein and Spradling 2007
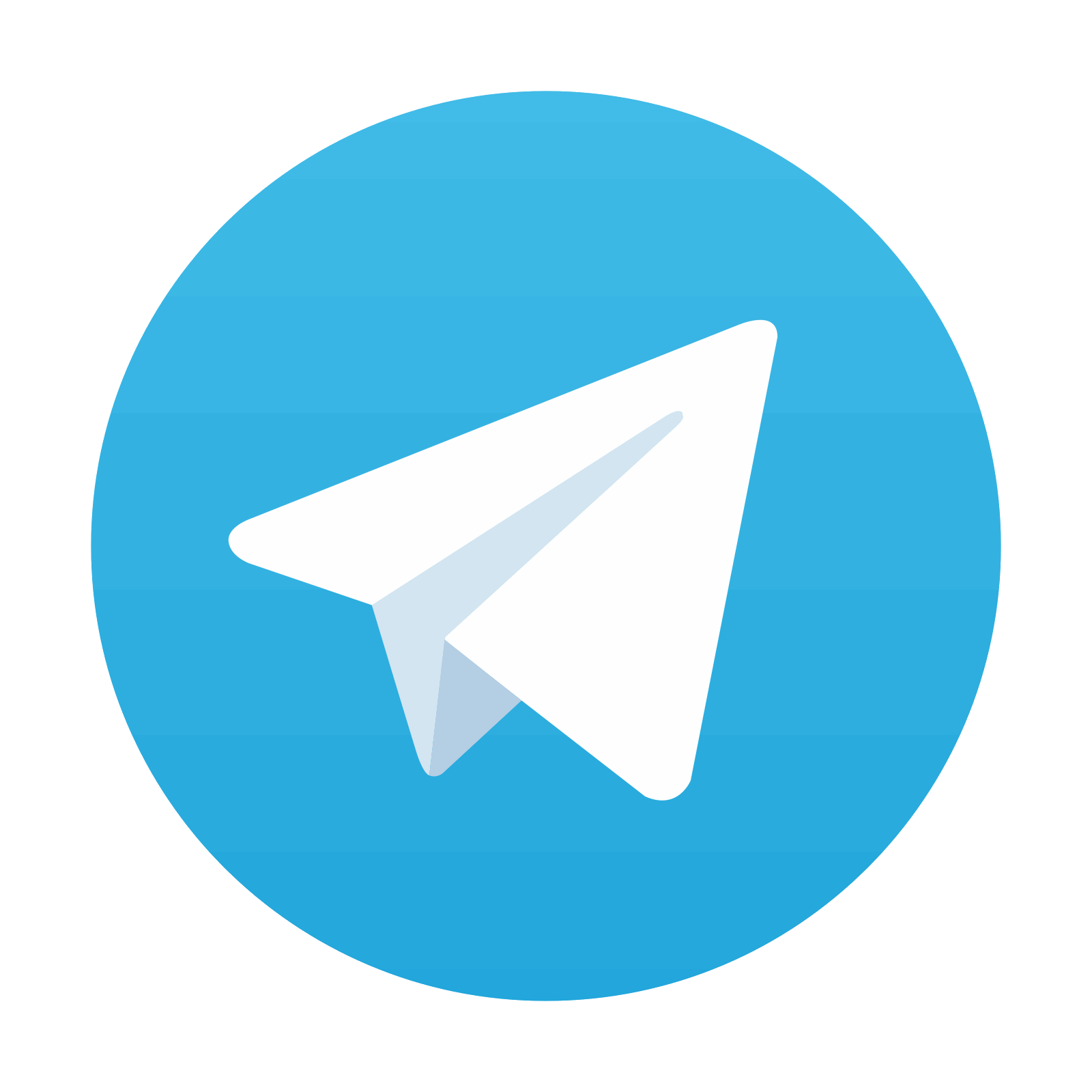
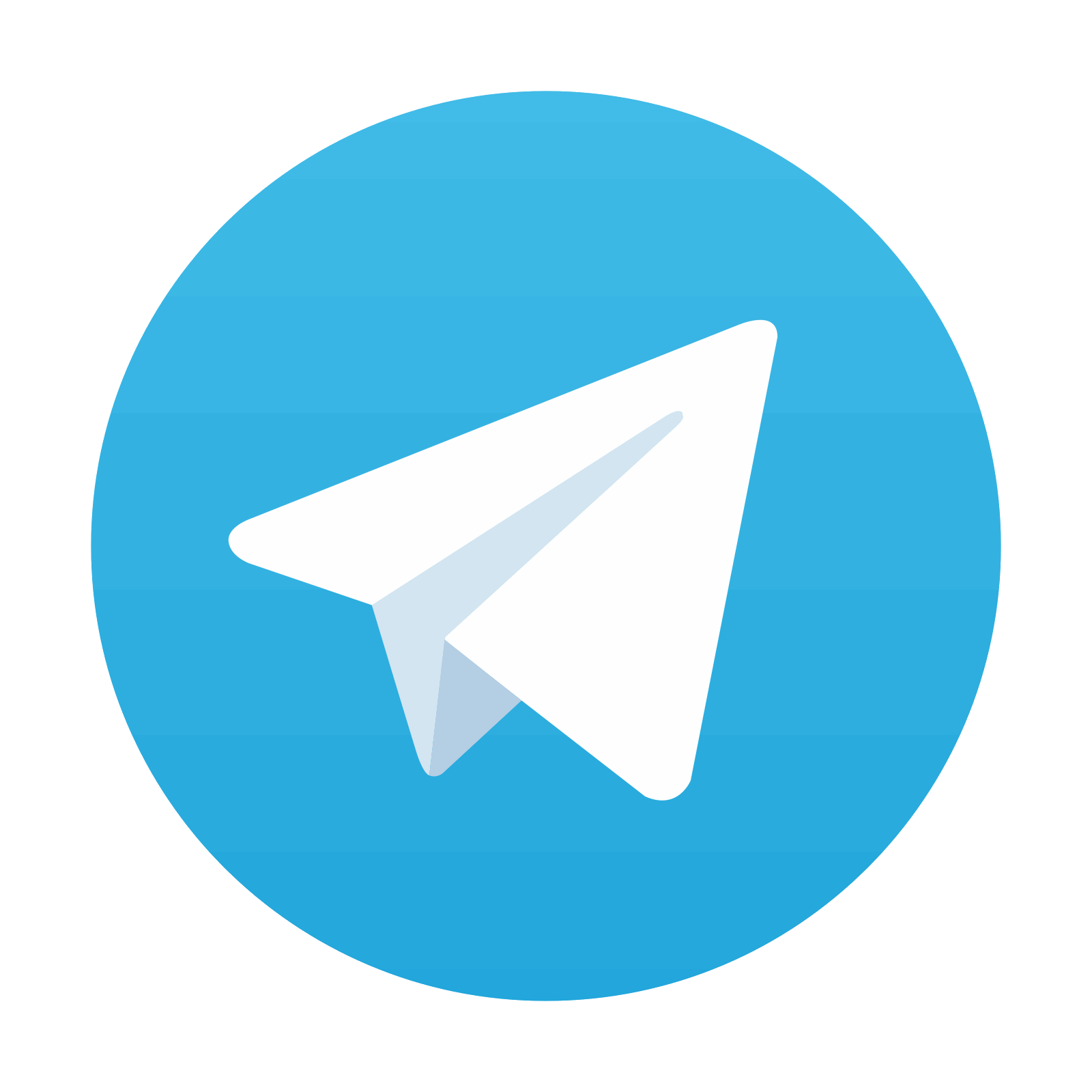
Stay updated, free articles. Join our Telegram channel

Full access? Get Clinical Tree
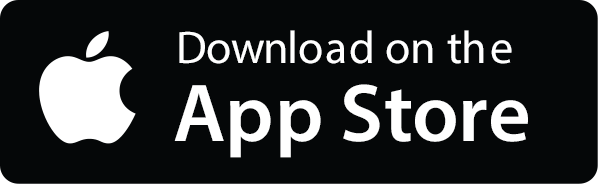
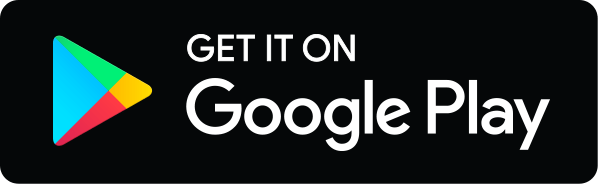