Karl Lintner KAL’IDEES S.A.S., Paris, France Peptides, proteins, and amino acids are often mislabeled and the terms applied as if they were interchangeable, yet they are different in their characteristics, uses, biological activities, and cosmetic potential [1]. The terms peptides and proteins are both somewhat ambiguous in the cosmetic domain and nomenclature: simple protein hydrolysates are often for marketing reasons called “peptides” (as for instance in Anonymous [2]), which are an undefined blend of protein fragments of unknown size, composition, activity. But the International Nomenclature for Cosmetic Ingredients (INCI) name of these products is “hydrolyzed wheat (or soy, fish, milk, yeast, potato..) protein. Synthetically engineered, specifically chosen peptide sequences on the other hand are pure analytically defined substances and will be the subject of this chapter. After defining peptides and proteins, the first part of the chapter discusses the specificities of these molecules and their physiologic, biological function, particularly in the skin; what can they do, what are the obstacles to their use in cosmetic products and how can these obstacles be overcome. In the five years since the previous edition of this book, a number of new such peptides have been studied, developed and used for cosmetic applications and both skin and hair care benefits. In the second part, concrete examples of peptides and some proteins, in particular for the “anti‐aging” sector, are discussed before concluding with the further outlook for the future of this ingredient category. It is important to understand the differences between amino acids, peptides, and proteins. Amino acids are the building blocks of which peptides and proteins are made. They are small molecules, with a molecular weight of 100–200 Dalton, characterized by the fact that both an amino group (NH2) and a carboxylic acid group (COOH) are attached to the central carbon atom (α‐carbon). This carbon atom with four valencies carries furthermore one hydrogen (except for the cyclic proline) and a side chain R that varies from amino acid to amino acid, side chains by which the different amino acids are distinguished (Figure 38.1). Given this narrow definition, we can imagine a theoretically unlimited number of alpha‐amino acids by simply varying the structure of the side chains, but only 20 (e.g. alanine, proline, tyrosine, histidine, phenylalanine, lysine, glutamine …) are incorporated into peptides and proteins via the genetic code. With few exceptions, these amino acids in isolation have no specific intrinsic biological activity. Within cells, they exist in a pool from which they can be called upon to make peptides and proteins or, sometimes, biogenic amines, such as serotonin or dopamine. In the upper layers of the skin, they are part of the natural moisturizing factor (NMF) where they participate in the water holding capacity of the skin contributing to both osmolytic and hygroscopic properties. The result of linking two or more amino acids in a linear chain via an amide bond between the carboxyl group of one and the amino group of the following amino acid is called a peptide, when the length of the chain is less than approximately 100 amino acids, or a protein when the chain is longer. Figure 38.1 Phenylalanine, one of the 20 proteinogenic amino acids. The “side chain” which is characteristic of each amino acid (here a phenyl group) is shown in the box. The general terminology uses prefixes to describe the type of a peptide. For example, when the peptide is made of two amino acids, such as tyrosine and arginine written as Tyr‐Arg, it is called a dipeptide. Three amino acid combinations yield a tripeptide, four amino acid combinations yield a tetrapeptide, etc. “Oligo” stands for a “few” so that oligopeptides can have 2 to ≈20 amino acids linked in a chain. The term polypeptide is used to mean many amino acids in a chain, although these latter distinctions are not strict and not governed by official rules. The most important characteristic of a peptide, besides its length determined by the number of amino acids in the chain, is its sequence. The sequence is the precise order in which the various amino acids are linked together. Both glycyl‐histidyl‐lysine and glycyl‐lysyl‐histidine are tripeptides, composed of the three amino acids glycine, histidine, and lysine. However, the fact that these amino acids are linked in the Gly‐His‐Lys sequence in the former and in Gly‐Lys‐His sequence in the latter is crucial. The former peptide, usually abbreviated GHK, stimulates collagen synthesis in fibroblasts [3]; the latter GKH stimulates lipolysis in adipocytes [4]. The primary biological function of most peptides in animal including human bodies is to convey a biochemical message from place A (a secretory gland) in the body to place B (e.g. a target cell or specific receptor). This allows for effective communication between different organs and structures in the organism (see below). A peptide chain of more than approximately 100 amino acids is usually termed a protein. However, interleukins, cytokines, and interferon are also sometimes referred to as peptides, even though they possess a much higher molecular weight. Sometimes the distinction between the two categories relies more on the function of the molecule rather than the size. Proteins can be categorized by their function, roughly into the following: Proteins with individual molecular mass of hundreds of thousands of Daltons often auto‐assemble into large structures with very complex mechanisms of activity. Peptides perform many important biologic signal (hormonal) functions. The word hormone – from Greek, simply meaning messenger – applies to various classes of substances (steroids, peptides, biogenic amines…). All messenger molecules, including certain peptides, act in similar fashion: some disturbance, either internal or external, leads to the release of a small amount of peptide in a cell, blood, gland, or in some other organ. The peptide then travels in the body until it interacts with a target receptor either on the cellular surface or within the cell nucleus after having penetrated the cell wall. This interaction triggers further activity at the site, destined to respond and to correct the initial disturbance. This mechanism of action is usually characterized by three items: Well‐known biological activities of peptide hormones in the human body are, for instance: regulation of blood sugar concentration (insulin); blood pressure regulation (angiotensin, bradykinin, calcitonin gene‐related peptide [CGRP]); lactation and birthing (oxytocin); diuresis (vasopressin); pain repression (endorphins, enkephalin); tanning (α‐MSH); radical scavenging (glutathione [GSH]); other peptides include vasointestinal peptide (VIP), substance P, neurotensin, luteotropin, and hundreds more. Peptides with various bioactivities have been identified in all organs of our body and the skin is no exception. An increasing volume of research is devoted to the understanding of peptide functions in the skin and consequently to find cosmetic applications of this huge category of bioactive substances. As this section’s term of “anti‐aging” is not clearly defined, it shall be interpreted here in a rather broad way to represent anything that helps the skin look younger. Hence we shall discuss, in the subsequent paragraphs, a variety of cosmetic skin care activities for which peptides have been developed and used with success, such as antioxidant peptides, tissue repair peptides, skin whitening or tanning peptides, soothing and neuromodulating peptides, hair growth controlling peptides, and mention only in passing lipid metabolism mediating peptides, moisturizing and barrier repair peptides and others. These examples are, of course, far from being exhaustive. A few words about potential pitfalls are, nevertheless, in order first. The incorporation of peptides into various galenic forms of skin care products can be challenging. Some of the hurdles confronted with peptide formulation include skin penetration, stability, toxicity, analysis, and cost. The stratum corneum (SC) is not the primary target for peptides, as they need viable, living skin to receive their message. It is necessary for a peptide to cross the cutaneous barrier in order to reach the viable epidermis (keratinocytes), the basal layer (melanocytes, nerve cell endings), the dermis (fibroblasts), and even the hypodermis (adipocytes). Even small peptide molecules, such as the dipeptide carnosine, are too hydrophilic and electrically charged to penetrate easily any further than the first or second layer of the SC. Lintner and Peschard [5] have shown that the attachment of a lipophilic chain (fatty acid of sufficient length) to carnosine can increase the penetration rate by a factor of 100 or more. Similar effects were confirmed by Leroux et al. [4]. Goebel et al. [6] have further studied the penetration behavior of this molecule and describe various techniques of formulation to overcome the problem. This technique of vectorizing the peptides has its limits because the longer the peptide chain, the less penetration the fatty acid will produce. The larger the peptide (beyond six or seven amino acids), the less likely it is to reach the deeper layers of the skin. Thus, the long peptide sequences of CGRP, proopiomelanocortin (POMC), epidermal growth factor (EGF), other chemokines, and similar structures of potential cosmetic interest do not function well as active ingredients in cosmetic formulas. Another limitation of this technique is the possible interference of biologic activity by acylation of the peptide. The N terminal ionic charge may be of importance for triggering effects at the target site or otherwise interfering with the peptide’s properties. For example, the antioxidant activity of carnosine turns into pro‐oxidant activity when the peptide is modified to become palmitoyl‐carnosine. Recent research has discovered alternative ways of increasing transcutaneous delivery, using amphiphilic cell penetration peptides (CPPs) [7], attaching a poly‐arginine chain to the peptide [8], or designing hyaluronic acid (HA) conjugates [9] to help molecules penetrate the SC. Liposome formulations may also help carry the peptide through the barrier, but little if anything has been published in this respect. How to help a peptide to reach its dermal targets must be investigated and tested case by case. Peptides have a reputation of limited chemical stability. While it is true that in aqueous environments, such as those frequently encountered in cosmetic applications, some degree of hydrolysis may occur, experience has shown that the right choice of excipients and stabilizers can help overcome this obstacle [10], the more so as the apparent instability (disappearance of the peptide from the cream) is often an analytically based artifact. The above‐mentioned long‐chain acylated lipopeptides have a tendency to self‐associate and to form gels which simply pose a challenge to the analytical chemist [11] but are not inactivated by such gelling. Detecting the presence of a peptide in a formulation 6–12 months after product manufacture can be difficult when the peptide is present in micromolar or lower concentrations (p.p.m. level). Special analytical techniques, such as derivatization, mass spectrometry, and fluorescence spectrometry, have to be individually developed for each peptide. This is not always possible and/or very costly and sometimes proves an insurmountable hurdle. A recent paper by Chirita et al. [12] demonstrates nevertheless both the possibility of detecting 3–5 ppm of a pentapeptide in a complex cream matrix and the proven long‐term stability of the substance. Generally, the smaller the peptide, the less likely it is to show untoward effects. Peptides, in contrast to proteins, are hardly big enough to elicit allergic reactions, but specific undesirable cellular effects may occur with unknown sequences. It is advisable to use peptides with a biomimetic amino acid sequence, as the likelihood of toxicity is close to nil when the peptide is – almost – identical to human peptides of known safety status. Nevertheless, proper safety evaluation of newly developed peptides, especially if the peptide is modified by acylation or esterification, is necessary. Over the ≈30 years of using such peptides in cosmetic products, to my knowledge, not a single instance of observed undesirable effects has been attributed to these peptides. Peptides of defined sequence and high purity (>90%) are relatively expensive to produce; although extraction from some protein hydrolysates is theoretically possible if even more costly, most peptides used in cosmetic applications are synthetic (i.e. made in a step‐by‐step process from the individual amino acid building blocks). It is noteworthy that the amino acids themselves are frequently of natural plant or fermentation origin. However, the very high potency of the peptides compensates for their cost and makes it possible to employ them at efficient level in all types of skin care formulas, because they are often used at the one‐digit p.p.m. level (0,0001–0009%) in finished cosmetics. Therefore, in spite of these formulation challenges, peptides have become widely used active ingredients for anti‐aging skin care products, discussed next. The INCI dictionary contains some 1400 synthetic peptides with numerous claimed activities and benefits. We will discuss a short but representative selection of such peptides. This tripeptide GSH is one of the “oldest” members of the peptide family, with respect to its discovery, analysis, and confirmatory synthesis. It contains an – SH bearing cysteine amino acid which confers antioxidant activity to the molecule (Figure 38.2). The level of GSH concentration in the body decreases notably with age, which may be a cause and a symptom of aging both at the same time [13]. Besides affording this protective, antioxidant activity, for which there is in vitro but very little documented clinical evidence of skin benefits (for a medical study, see Enomoto et al. [14]). GSH may also have so‐called “skin whitening” effects, as described by Villarama and Maibach [15]. Carnosine has been proven to scavenge reactive oxygen species (ROS) formed from peroxidation of cell membrane fatty acids during oxidative stress. Carnosine is also shown to be useful to counter the effects of glycation (the nonenzymatic binding of sugars to proteins) which leads to cytotoxic advanced glycation endproducts (AGEs). A new derivative of azelaic acid and histidine (azeloyl‐tetrapeptide 23) also possesses antioxidant and strong anti‐glycation activity [16]. Nagai et al. [17] showed that carnosine might also promote wound healing, at least indirectly, as exogenous carnosine is degraded by carnosinase into β‐alanine and ultimately histamine. Whereas β‐alanine was found to stimulate the biosynthesis of nucleic acids and collagen, histamine is considered to enhance the process of wound healing by stimulating effusion at the initial stage of inflammation. This example illustrates the economical side of nature using both the original substance (carnosine) and both its metabolic fragments for concurring purposes, a mechanism found particularly often with peptides. Figure 38.2 Glutathione (γ‐glutamyl‐cysteinyl‐glycine). One of the most popular claims of anti‐aging concerns the repair or restoration of the extracellular matrix (ECM) of the skin, mainly to reduce the appearance of wrinkles and other signs of photoaging. The term matrikines is used to describe naturally occurring fragments of matrix macromolecules endowed with stimulatory, tissue repair activity [18]. Schematically and very simplified, this matrikine concept of recycling breakdown fragments of macromolecules for triggering repair activity is illustrated in Figure 38.3. The concept is valid not only for proteins and peptide fragments but also for poly‐ and oligosaccharides, where the term glycokine applies. The best‐known matrikine peptide used in skin care is the pentapeptide Palmitoyl‐lysyl‐threonyl‐threonyl‐lysyl‐serine (Pal‐KTTKS), derived from the shortest pro‐collagen I fragment capable of stimulating collagen synthesis in fibroblasts (Katayama et al. [19]). A DNA array study on this molecule indicated that mostly genes implicated in the wound healing process were upregulated in cells incubated with the peptide. Furthermore, the palmitoylated peptide stimulates not only the synthesis of collagen I, but also of collagen IV, fibronectin, and glycosaminoglycanes in monolayer culture of normal and aged human fibroblasts and in full thickness skin [20]. This peptide was tested in vehicle‐controlled clinical trials where it proved to thicken the skin, improve the epidermal–dermal junction, and macroscopically reduce fine lines and wrinkles [20–22]. Hajem et al. [23] describe the naturally occurring Ac‐Ser‐Asp‐Lys‐Pro tetrapeptide as an angiogenic factor that contributes to repair of cutaneous injuries. A substantial amount of work has gone into studying the activities of this peptide at the level of in vitro mechanisms. The tetrapeptide Arg‐Gly‐Asp‐Ser (RGDS), a sequence found within the fibronectin structure responsible for the binding affinity of this protein to collagen and to cell membranes, is able to help cells migrate during the wound healing process. A cyclic RGD peptide, able to bind to integrin receptors was tested in a clinical study and found to possess anti‐wrinkle properties [24]. The peptide Pal‐Gly‐Gln‐Pro‐Arg (Pal‐GQPR) is a fragment of the natural circulating protein IgG and stimulates macromolecule synthesis in cell culture but also contributes to the reduction of basal and UV‐induced IL‐6 release in keratinocytes and fibroblasts (Table 38.1), which leads to improved skin firmness in vivo. The tripeptide Gly‐His‐Lys, found in different parts of broken‐down collagen and in some serum proteins, also stimulates collagen synthesis in human skin fibroblasts, as found by Maquart et al. [3]. In its palmitoylated form (Pal‐GHK), it can mimic the effects of retinoic acid [5]. The in vitro synergy between the tri‐ and tetrapeptide (Pal‐GHK + Pal‐GQPR) [25] led to an investigation of the combination in a clinical, vehicle controlled, blind study on 23 panelists. Twice daily application of an oil‐in‐water (O/W) emulsion containing 4 p.p.m. of Pal‐GHK and 2 p.p.m. of Pal‐GQPR against vehicle showed significant wrinkle reduction, an increase in skin firmness, and visible smoothing after 1–2 months. Measuring the Subepidermal Low Echogenic Band (SLEB: Figure 38.4) by ultrasound echography, Mondon et al. [26] were able to show, based on the strong correlation between age and the thickness of the SLEB described by Querleux et al. [27], a decrease in apparent age of the skin of ≈5 years in a panel of volunteers using the peptide containing creams for 2 months.
CHAPTER 38
Peptides and Proteins
Introduction
Definitions
Amino acids
Peptides
Proteins
Biological functions of peptides and proteins in the skin
Peptides
Obstacles to peptide use in cosmetic formulation
Skin penetration
Stability
Analysis
Toxicity
Cost
Antioxidant peptides
Glutathione (γ‐glutamyl‐cysteyl‐glycine)
Carnosine (β‐alanyl‐l‐histidine)
Tissue repair peptides
Matrikines
Stay updated, free articles. Join our Telegram channel

Full access? Get Clinical Tree
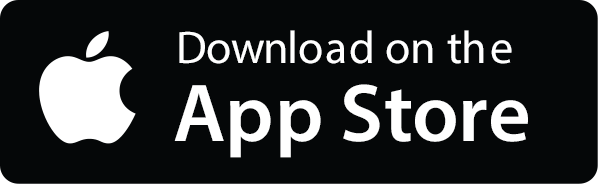
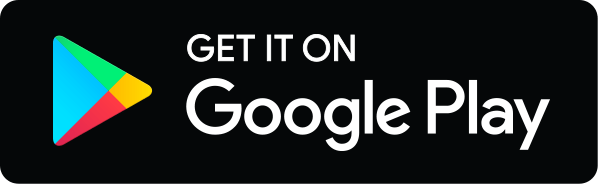